An Electrical Property That Opposes A Change In Current
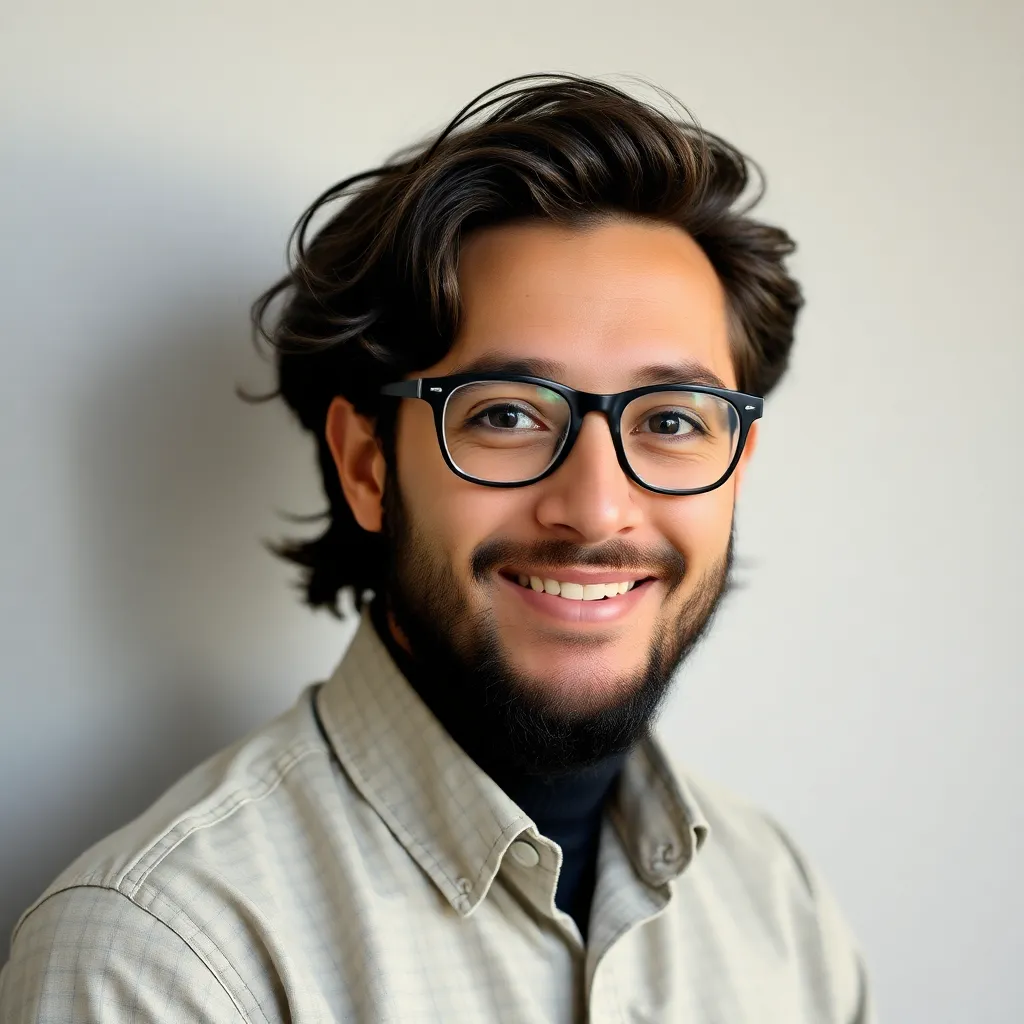
Juapaving
Apr 05, 2025 · 7 min read
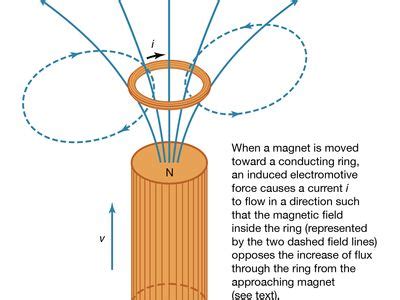
Table of Contents
Inductance: The Electrical Property that Opposes Change in Current
Inductance is a fundamental electrical property that describes an element's ability to oppose changes in electric current. It's a crucial concept in understanding how circuits behave, particularly in alternating current (AC) systems and electromagnetic applications. This comprehensive guide delves deep into the nature of inductance, its applications, and its role in various electrical phenomena.
Understanding Inductance: The Basics
At its core, inductance is the tendency of an electrical conductor to oppose a change in the electric current flowing through it. This opposition manifests as a voltage that is induced across the conductor, often referred to as a back electromotive force (back EMF). This back EMF acts in a direction that counteracts the change in current, whether that change is an increase or a decrease.
The primary cause of inductance lies in electromagnetism. When an electric current flows through a conductor, it generates a magnetic field around it. The strength of this magnetic field is directly proportional to the current. When the current changes, the magnetic field changes accordingly. This changing magnetic field, in turn, induces a voltage in the conductor itself, according to Faraday's Law of Induction. This induced voltage opposes the change in the current, hence the term "self-inductance".
The Unit of Inductance: The Henry
Inductance is quantified in henries (H). One henry is defined as the inductance that produces a back EMF of one volt when the current changes at a rate of one ampere per second. The henry is a relatively large unit, and smaller units like millihenries (mH) and microhenries (µH) are frequently used in practice.
Factors Affecting Inductance
Several factors influence the inductance of a conductor:
1. Number of Turns:
The inductance of a coil is directly proportional to the square of the number of turns. Doubling the number of turns in a coil quadruples its inductance. This is because a greater number of turns increases the magnetic field strength for a given current, resulting in a stronger back EMF.
2. Coil Geometry:
The physical shape and size of the coil significantly impact inductance. Longer coils generally have higher inductance than shorter coils, and coils with a larger cross-sectional area also exhibit higher inductance. The type of core material also plays a crucial role; a ferromagnetic core (like iron) significantly enhances the magnetic field and thus the inductance, compared to an air core.
3. Core Material:
The material of the core within the coil substantially affects the inductance. Ferromagnetic materials such as iron, nickel, and ferrite have high permeability, meaning they readily allow magnetic fields to pass through them. This significantly increases the magnetic field strength and, consequently, the inductance. Air-core inductors have much lower inductance.
4. Permeability of the Core Material:
Permeability (µ) is a measure of a material's ability to support the formation of a magnetic field. High permeability materials concentrate the magnetic field lines, leading to higher inductance. The permeability of the core material is a crucial factor in determining the overall inductance of the coil.
Types of Inductors
Inductors come in various forms, each designed for specific applications:
1. Air-Core Inductors:
These inductors have an air core, resulting in lower inductance compared to those with ferromagnetic cores. They are often used in high-frequency applications where the core's losses would be significant.
2. Iron-Core Inductors:
These inductors utilize iron cores to significantly increase inductance. However, iron cores introduce hysteresis and eddy current losses, limiting their application to lower frequencies.
3. Ferrite-Core Inductors:
Ferrite cores offer a good compromise between high permeability and low losses. They are widely used across a broader range of frequencies than iron-core inductors.
4. Toroidal Inductors:
These inductors have a doughnut-shaped core, which confines the magnetic field, minimizing leakage flux and improving efficiency. They exhibit high inductance for a given size.
Inductance in Circuits: Series and Parallel Connections
When inductors are connected in circuits, their combined inductance depends on the configuration:
1. Series Connection:
In a series connection, the total inductance (L<sub>total</sub>) is simply the sum of individual inductances:
L<sub>total</sub> = L<sub>1</sub> + L<sub>2</sub> + L<sub>3</sub> + ...
This assumes negligible magnetic coupling between the inductors.
2. Parallel Connection:
The total inductance of inductors connected in parallel is calculated using the reciprocal formula, similar to resistors in parallel:
1/L<sub>total</sub> = 1/L<sub>1</sub> + 1/L<sub>2</sub> + 1/L<sub>3</sub> + ...
Again, this assumes negligible magnetic coupling.
Mutual Inductance: The Interaction Between Inductors
When two or more inductors are placed close together, their magnetic fields interact, leading to a phenomenon called mutual inductance. Mutual inductance (M) describes the induced voltage in one inductor due to a changing current in another inductor. The magnitude of mutual inductance depends on the geometry of the coils, their relative orientation, and the permeability of the surrounding medium.
Mutual inductance is particularly important in transformers, where it facilitates the transfer of energy between two electrically isolated circuits.
Inductance and AC Circuits: Impedance and Phase Shift
In direct current (DC) circuits, the inductor acts as a short circuit once the current stabilizes. However, in alternating current (AC) circuits, the inductor exhibits impedance, which is the opposition to the flow of AC current. This impedance is directly proportional to the frequency (f) of the AC signal and the inductance (L):
Z<sub>L</sub> = jωL = j2πfL
Where 'j' is the imaginary unit, and ω is the angular frequency (ω = 2πf). The impedance is a complex quantity, indicating a phase shift between the voltage and current. In an inductor, the voltage leads the current by 90 degrees.
Applications of Inductors
Inductors play vital roles in a vast array of electrical and electronic applications:
1. Energy Storage:
Inductors can store energy in their magnetic fields. This energy can be released back into the circuit when the current decreases. This property is exploited in various applications, including switching power supplies and resonant circuits.
2. Filtering:
Inductors are essential components in filters used to separate signals based on their frequency. They effectively block high-frequency signals while allowing low-frequency signals to pass through. This is commonly used in power supplies to smooth out ripple voltage.
3. Tuning Circuits:
Inductors, in conjunction with capacitors, form resonant circuits that are used to select specific frequencies in radio receivers and transmitters. The resonant frequency is determined by the inductance and capacitance values.
4. Transformers:
Transformers utilize mutual inductance to transfer energy between two circuits without a direct electrical connection. This is crucial for power transmission and voltage transformation in many applications.
5. Sensors:
Inductors are used in various sensor applications to measure changes in magnetic fields or proximity. Changes in inductance can be used to detect the presence of objects or to measure displacement.
Troubleshooting Inductors
Faulty inductors can manifest in several ways:
- Open Circuit: A broken inductor will completely prevent current flow.
- Short Circuit: A shorted inductor will behave like a piece of wire, offering minimal impedance.
- Change in Inductance: Ageing, overheating, or physical damage can alter the inductance value, leading to malfunction in circuits.
Testing inductors often requires specialized equipment like an LCR meter, which measures inductance, capacitance, and resistance. Visual inspection for physical damage is also a crucial first step.
Conclusion: The Indispensable Role of Inductance
Inductance, as a fundamental electrical property, is essential for understanding and designing countless electrical and electronic systems. Its ability to oppose changes in current makes it crucial in applications ranging from energy storage and filtering to signal processing and power transmission. A thorough understanding of inductance, its characteristics, and its applications is critical for anyone working in electrical engineering or related fields. This article provides a comprehensive foundation for further exploration of this fascinating and indispensable aspect of electrical circuits.
Latest Posts
Latest Posts
-
Cannot Be Measured Ray Or Line Segment
Apr 06, 2025
-
Suppose You Are Walking Down A Street
Apr 06, 2025
-
How Many Feet Is 114 Inches
Apr 06, 2025
-
How To Factorize A Quadratic Equation
Apr 06, 2025
-
Negative 16 X Greater Or Equal Than Negative 48
Apr 06, 2025
Related Post
Thank you for visiting our website which covers about An Electrical Property That Opposes A Change In Current . We hope the information provided has been useful to you. Feel free to contact us if you have any questions or need further assistance. See you next time and don't miss to bookmark.