The Physical Expression Of An Organism's Genes
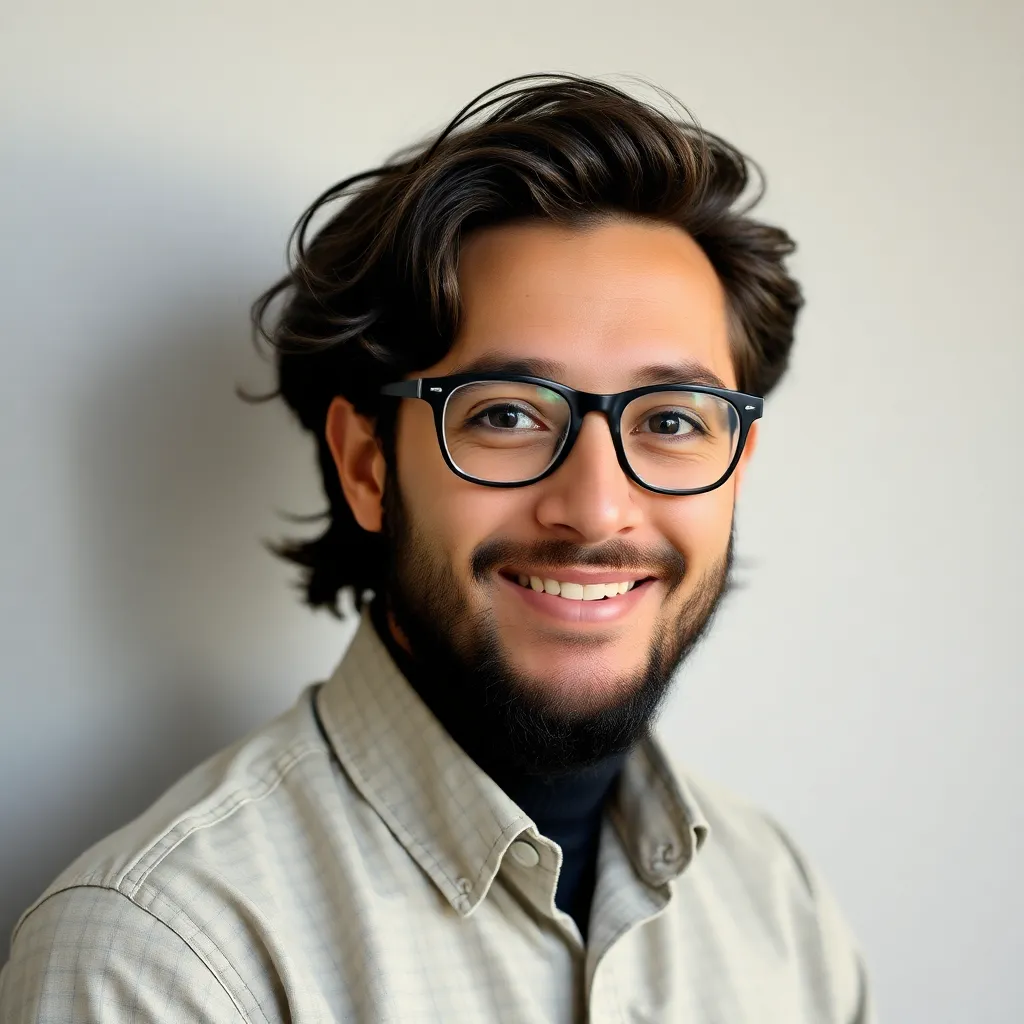
Juapaving
May 09, 2025 · 6 min read
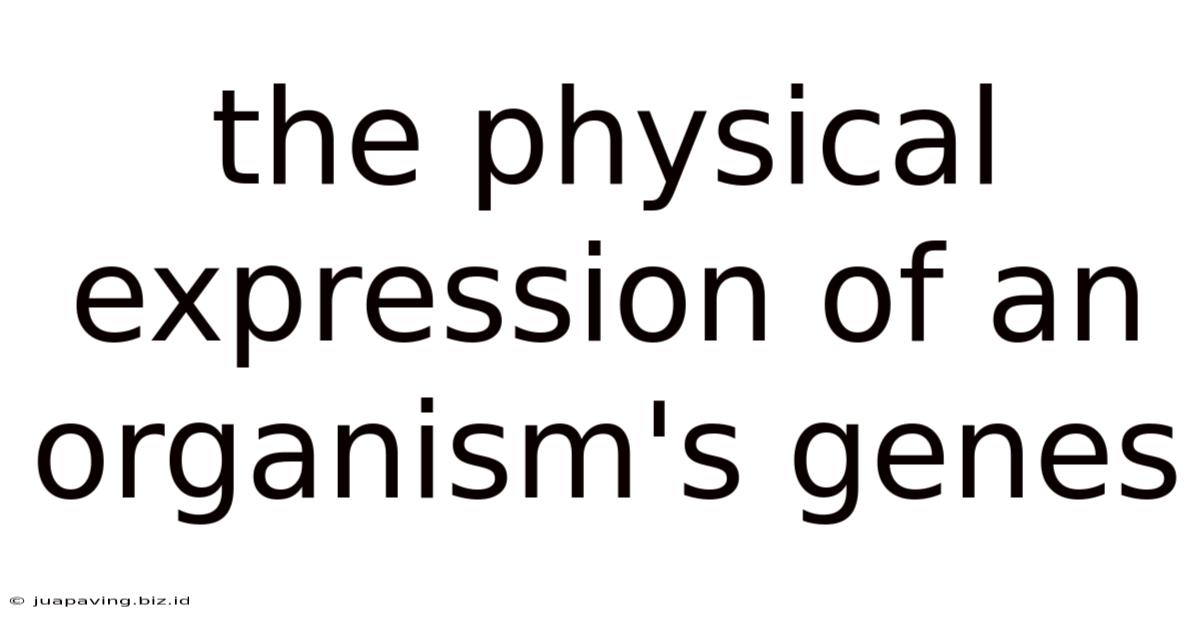
Table of Contents
The Physical Expression of an Organism's Genes: From Genotype to Phenotype
The intricate dance between genes and the physical characteristics of an organism, known as the genotype-phenotype relationship, is a fundamental concept in biology. This article delves deep into the fascinating world of how an organism's genetic blueprint translates into its observable traits, exploring the complex mechanisms that bridge the gap between DNA and the physical expression of life. We will examine various aspects, including gene expression, environmental influences, and the implications of this relationship for understanding evolution, disease, and the diversity of life on Earth.
Understanding the Basic Concepts
Before diving into the intricacies of gene expression, it's crucial to define key terms. Genotype refers to the complete set of genes an organism possesses, its genetic makeup encoded within its DNA. Phenotype, on the other hand, encompasses all the observable characteristics of an organism, including its physical traits (morphology), physiological properties, and behavior. The phenotype is the culmination of the genotype interacting with the environment.
Genes, the functional units of heredity, are specific sequences of DNA that provide instructions for building proteins. These proteins, in turn, perform a vast array of functions within the cell and organism, ultimately shaping the phenotype. The relationship is not simply a one-to-one correspondence; many genes contribute to a single trait, and a single gene can influence multiple traits (pleiotropy).
The Central Dogma of Molecular Biology: Transcription and Translation
The process of translating genetic information from DNA into a functional protein is described by the central dogma of molecular biology. This process comprises two major steps:
Transcription: DNA to RNA
Transcription is the first step in gene expression. It involves the synthesis of a messenger RNA (mRNA) molecule from a DNA template. The DNA double helix unwinds, and an enzyme called RNA polymerase reads the DNA sequence, creating a complementary RNA strand. This mRNA molecule carries the genetic code from the nucleus (in eukaryotes) to the ribosomes, the protein synthesis machinery of the cell.
Translation: RNA to Protein
Translation is the second step, where the mRNA sequence is decoded into a sequence of amino acids, the building blocks of proteins. This process occurs in ribosomes, which read the mRNA sequence in three-nucleotide units called codons. Each codon specifies a particular amino acid. Transfer RNA (tRNA) molecules, carrying specific amino acids, recognize the codons and deliver the appropriate amino acids to the ribosome, forming a growing polypeptide chain. Once the polypeptide chain is complete, it folds into a three-dimensional structure, becoming a functional protein.
Beyond the Central Dogma: Regulatory Mechanisms
While the central dogma provides a fundamental framework, the process of gene expression is far more nuanced and regulated. Numerous mechanisms control which genes are expressed, when, and to what extent. These regulatory mechanisms are critical for ensuring that the organism develops and functions correctly. Some key regulatory mechanisms include:
Transcriptional Regulation: Controlling the Initiation of Transcription
Transcriptional regulation is the primary mechanism controlling gene expression. It involves various factors, including:
- Promoters: Regions of DNA upstream of the gene that bind RNA polymerase, initiating transcription. The strength of the promoter influences the rate of transcription.
- Enhancers and Silencers: DNA sequences that can increase (enhancers) or decrease (silencers) the rate of transcription, even at considerable distances from the gene.
- Transcription Factors: Proteins that bind to specific DNA sequences, either promoting or inhibiting the binding of RNA polymerase. These factors can be influenced by various environmental and developmental signals.
- Epigenetic Modifications: Chemical modifications to DNA and histone proteins that package DNA, altering the accessibility of genes to the transcriptional machinery. These modifications can be inherited and play a significant role in gene regulation.
Post-Transcriptional Regulation: Modifying mRNA and Protein
Regulation doesn't stop after transcription. Many mechanisms regulate gene expression after mRNA has been synthesized, including:
- RNA Splicing: In eukaryotes, the primary mRNA transcript contains introns (non-coding sequences) and exons (coding sequences). Splicing removes the introns and joins the exons to create the mature mRNA. Alternative splicing can produce different mRNA molecules from the same gene, leading to different protein isoforms.
- mRNA Stability and Degradation: The lifespan of mRNA molecules varies, influencing the amount of protein produced. The stability of mRNA is regulated by various factors.
- Translational Regulation: The rate of protein synthesis can be controlled at the ribosome. Initiation factors, regulatory proteins, and the availability of amino acids all play a role.
- Post-Translational Modification: After synthesis, proteins can undergo modifications such as phosphorylation, glycosylation, or cleavage, influencing their activity, localization, and lifespan.
Environmental Influence on Gene Expression
The environment plays a significant role in shaping the phenotype. Environmental factors can influence gene expression in several ways:
- Temperature: Many organisms exhibit temperature-dependent gene expression, resulting in phenotypic plasticity, such as changes in coat color or enzyme activity in response to temperature variations.
- Nutrition: Dietary factors can influence gene expression, impacting growth, development, and susceptibility to disease.
- Stress: Environmental stressors such as drought, pollution, or pathogen infection can alter gene expression, triggering responses to cope with these challenges.
- Light: Photoperiod (day length) influences gene expression in many organisms, regulating processes such as flowering in plants and reproduction in animals.
Epigenetics: Heritable Changes in Gene Expression
Epigenetics refers to heritable changes in gene expression that do not involve alterations to the underlying DNA sequence. These changes are often induced by environmental factors and can have long-lasting effects on the organism. Key epigenetic mechanisms include:
- DNA Methylation: The addition of a methyl group to cytosine bases in DNA, often silencing gene expression.
- Histone Modification: Chemical modifications to histone proteins that package DNA, altering chromatin structure and gene accessibility.
The Implications of Genotype-Phenotype Relationships
Understanding the genotype-phenotype relationship has profound implications across various fields:
- Medicine: Many diseases are caused by genetic mutations or disruptions in gene expression. Knowing how genes contribute to disease helps in developing diagnostic tools, therapies, and preventative measures.
- Agriculture: Understanding the genetic basis of desirable traits in crops and livestock allows breeders to develop high-yielding, disease-resistant, and nutritious varieties through selective breeding or genetic engineering.
- Evolutionary Biology: The genotype-phenotype relationship is central to evolutionary theory. Natural selection acts on phenotypes, but the underlying genetic changes drive evolutionary change.
- Conservation Biology: Understanding how genetic diversity affects the ability of populations to adapt to environmental change is crucial for conservation efforts.
Conclusion
The physical expression of an organism's genes is a multifaceted process involving intricate molecular mechanisms and environmental influences. The journey from genotype to phenotype is a complex interplay of transcription, translation, regulatory networks, and epigenetic modifications. By understanding these processes, we gain invaluable insights into the diversity of life, the causes of diseases, and the mechanisms of evolution. Further research into this field will undoubtedly reveal more about the remarkable relationship between an organism's genes and its observable characteristics, leading to breakthroughs in medicine, agriculture, and our understanding of the living world.
Latest Posts
Latest Posts
-
How To Find Coordinates Of Circumcenter
May 11, 2025
-
6 012 As A Fraction In Simplest Form
May 11, 2025
-
Which Graph Represents Y 3 Sqrt X
May 11, 2025
-
To Which Domain Do Humans Belong
May 11, 2025
-
What Percentage Is 28 Out Of 35
May 11, 2025
Related Post
Thank you for visiting our website which covers about The Physical Expression Of An Organism's Genes . We hope the information provided has been useful to you. Feel free to contact us if you have any questions or need further assistance. See you next time and don't miss to bookmark.