Phases Of Action Potential In Heart
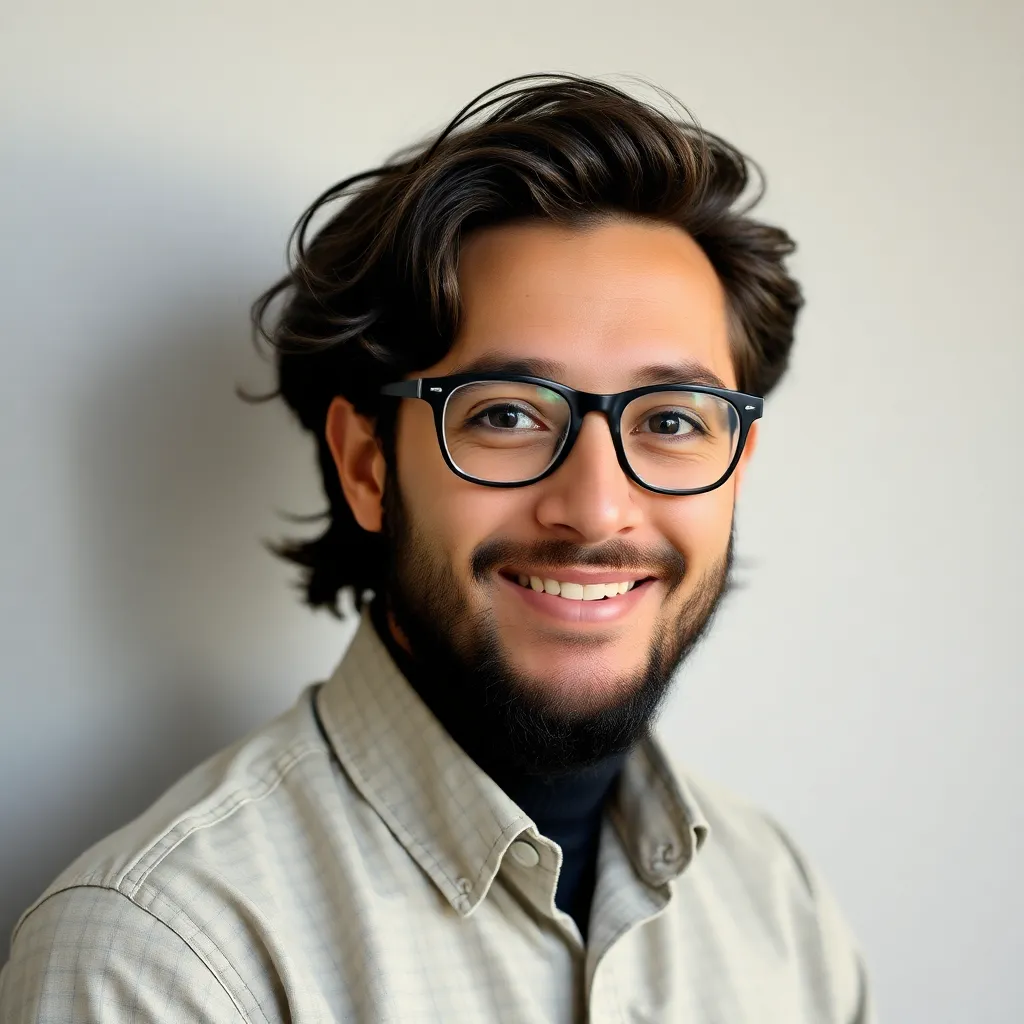
Juapaving
Apr 05, 2025 · 7 min read
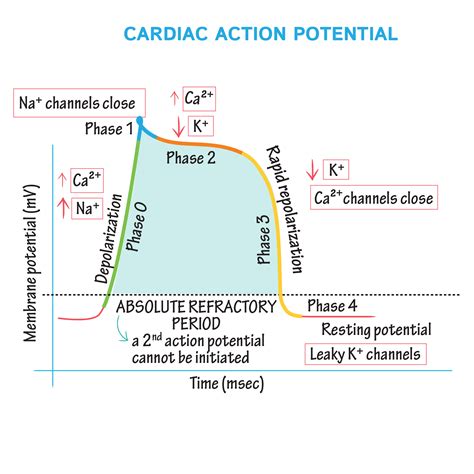
Table of Contents
Phases of the Action Potential in the Heart: A Comprehensive Guide
The human heart, a tireless muscle, beats rhythmically throughout our lives, propelled by the intricate dance of electrical signals known as action potentials. Understanding the phases of these action potentials is crucial to grasping the complexities of cardiac function, arrhythmias, and the effects of various drugs on the heart. This article will delve deeply into the phases of the cardiac action potential, exploring the ionic mechanisms that underlie each stage and highlighting the differences between various cardiac cell types.
The Cardiac Action Potential: An Overview
Unlike the brief action potentials seen in neurons, cardiac action potentials are significantly longer, lasting hundreds of milliseconds. This extended duration is essential for the coordinated contraction and relaxation of the heart muscle, enabling efficient pumping of blood throughout the body. The prolonged action potential is a direct result of the unique properties of ion channels found in cardiac myocytes (heart muscle cells).
The heart comprises various cell types, each exhibiting unique action potential characteristics. The most significant distinction is between the action potentials of working myocytes (found in the atria and ventricles, responsible for contraction) and pacemaker cells (located in the sinoatrial (SA) node, atrioventricular (AV) node, Bundle of His, and Purkinje fibers, responsible for initiating and conducting the electrical impulse). While both share some similarities, their key differences drive the heart's rhythmic beating. This article will primarily focus on the action potential of ventricular myocytes, as they represent the most common type of working myocyte and display the most complex phases.
Phases of the Ventricular Myocyte Action Potential
The ventricular myocyte action potential is typically divided into five phases: Phase 0, Phase 1, Phase 2 (plateau phase), Phase 3, and Phase 4. Let's explore each in detail:
Phase 0: Rapid Depolarization
This phase marks the beginning of the action potential. It is characterized by a rapid influx of sodium ions (Na⁺) into the cell through voltage-gated fast sodium channels. These channels open when the membrane potential reaches a threshold level, typically around -65 mV. The massive influx of positively charged sodium ions causes a dramatic and swift depolarization of the cell membrane, resulting in a sharp upward spike in the action potential waveform. The membrane potential rapidly rises to approximately +30 mV. This rapid depolarization is responsible for the initial electrical activation of the myocyte, triggering the process of contraction. The steepness of this phase is crucial for the coordinated and rapid spread of excitation through the heart muscle.
Phase 1: Early Repolarization
Following the rapid depolarization of Phase 0, Phase 1 initiates a brief period of early repolarization. This phase is characterized by a slight decrease in membrane potential. It's primarily driven by the inactivation of fast sodium channels and the opening of transient outward potassium channels (Ito). These potassium channels allow a small efflux of potassium ions (K⁺) out of the cell, counteracting the inward sodium current and leading to a small, initial repolarization. This phase is relatively short-lived and marks the transition to the plateau phase. The brevity of Phase 1 helps to ensure a smooth transition into the sustained depolarization of the plateau phase.
Phase 2: Plateau Phase
This is a unique and crucial phase of the cardiac action potential, setting it apart from action potentials in other excitable tissues. The plateau phase is characterized by a relatively sustained depolarization at a plateau potential near 0 mV for an extended period (hundreds of milliseconds). This prolonged depolarization is due to a delicate balance between two major ionic currents:
-
Inward calcium current (ICa): Voltage-gated L-type calcium channels open during this phase, allowing a slow but sustained influx of calcium ions (Ca²⁺) into the cell. This inward calcium current is crucial for initiating muscle contraction. The influx of Ca²⁺ triggers the release of even more calcium from the sarcoplasmic reticulum (SR), the intracellular calcium store, via calcium-induced calcium release (CICR), amplifying the calcium signal and resulting in a powerful contraction.
-
Outward potassium current (IK): Several types of potassium channels contribute to the outward potassium current during the plateau phase. These channels allow potassium ions to leave the cell, counteracting the inward calcium current. The balance between the inward calcium current and the outward potassium current maintains the plateau potential. Any imbalance in these currents can lead to significant changes in the duration of the action potential and consequently, the duration of the cardiac muscle contraction.
Phase 3: Rapid Repolarization
This phase marks the final repolarization of the ventricular myocyte. It is characterized by a rapid decrease in membrane potential, returning the cell to its resting state. The primary ionic mechanism driving this phase is the increased outward potassium current. As the calcium channels slowly inactivate, the outward potassium current becomes dominant, leading to a rapid efflux of potassium ions from the cell. This repolarization phase restores the negative membrane potential necessary for the cell to become excitable again, allowing another action potential to be initiated. The swiftness of this repolarization is critical for the heart to relax effectively between contractions and prepare for the next cycle.
Phase 4: Resting Membrane Potential
Once the cell has fully repolarized, it enters Phase 4, the resting membrane potential. This is the steady-state membrane potential of the cell before the next action potential is initiated. The membrane potential remains relatively stable at around -90 mV. During this phase, the sodium-potassium pump actively transports sodium ions out of the cell and potassium ions into the cell, maintaining the concentration gradients necessary for the generation of subsequent action potentials. This phase is crucial in ensuring the readiness of the cardiomyocyte for the initiation of the next electrical impulse. The stability of this phase is essential for maintaining the rhythm of the heartbeat.
Differences in Action Potentials Across Cardiac Cell Types
While the ventricular myocyte action potential is described above, other cardiac cells show variations in their action potentials:
-
Atrial Myocytes: Atrial myocytes have shorter action potentials compared to ventricular myocytes. They have a less pronounced plateau phase and a faster repolarization.
-
Pacemaker Cells (SA and AV Nodes): Pacemaker cells do not have a true resting membrane potential. Their membrane potential slowly depolarizes, reaching a threshold potential that triggers an action potential spontaneously. This automaticity is the basis of the heart's inherent rhythmicity. Their action potentials lack a clear Phase 1 and have a much slower Phase 0 due to the presence of slower calcium channels. The slow depolarization of Phase 4, driven by the “funny current” (If), is a defining characteristic of pacemaker cells.
-
Purkinje Fibers: Purkinje fibers have a rapid rate of conduction, facilitating the rapid spread of excitation through the ventricles. They display a fast upstroke (Phase 0) similar to ventricular myocytes, but their plateau phase is shorter and their repolarization is faster.
Clinical Significance
Understanding the phases of the cardiac action potential is critical in various clinical settings. Disruptions in the normal functioning of ion channels or imbalances in ionic currents can lead to various cardiac arrhythmias. For instance:
-
Long QT Syndrome: This condition is characterized by a prolonged QT interval on the electrocardiogram (ECG), reflecting a prolonged ventricular action potential. This can increase the risk of life-threatening arrhythmias such as torsades de pointes. Mutations in genes encoding ion channels are often implicated in this syndrome.
-
Brugada Syndrome: This is associated with changes in sodium channels and leads to abnormal repolarization, increasing the risk of ventricular fibrillation.
-
Heart Failure: Alterations in calcium handling and changes in the action potential duration contribute to the impaired contractility seen in heart failure.
-
Drug Effects: Many antiarrhythmic drugs target specific ion channels to modify the action potential, thereby influencing the heart's rhythm and contractility. Understanding the specific effects of these drugs on different phases of the action potential is essential for their safe and effective use.
Conclusion
The phases of the cardiac action potential are a complex and tightly regulated process essential for maintaining normal heart function. The intricate interplay of various ion channels and the specific characteristics of each phase in different cardiac cell types determine the rhythm and efficiency of the heart. A deep understanding of these phases is crucial for clinicians to diagnose, manage, and treat various cardiac arrhythmias and other heart conditions. Future research in this area will likely continue to reveal finer details of this vital process, contributing to advancements in the diagnosis and treatment of cardiovascular disease. The detailed knowledge of these phases also plays a significant role in drug development aimed at targeting specific ionic currents to restore or improve cardiac function. Continued research promises further advancements in our understanding of this fundamental process.
Latest Posts
Latest Posts
-
Buffer Region On A Titration Curve
Apr 06, 2025
-
What Is The Relationship Between Kinetic And Potential Energy
Apr 06, 2025
-
Conversion Of Mechanical Energy To Electrical Energy
Apr 06, 2025
-
Is A Rectangle A Regular Polygon
Apr 06, 2025
-
5 Letter Words Starting With R I
Apr 06, 2025
Related Post
Thank you for visiting our website which covers about Phases Of Action Potential In Heart . We hope the information provided has been useful to you. Feel free to contact us if you have any questions or need further assistance. See you next time and don't miss to bookmark.