Compressions And Rarefactions Are Characteristics Of
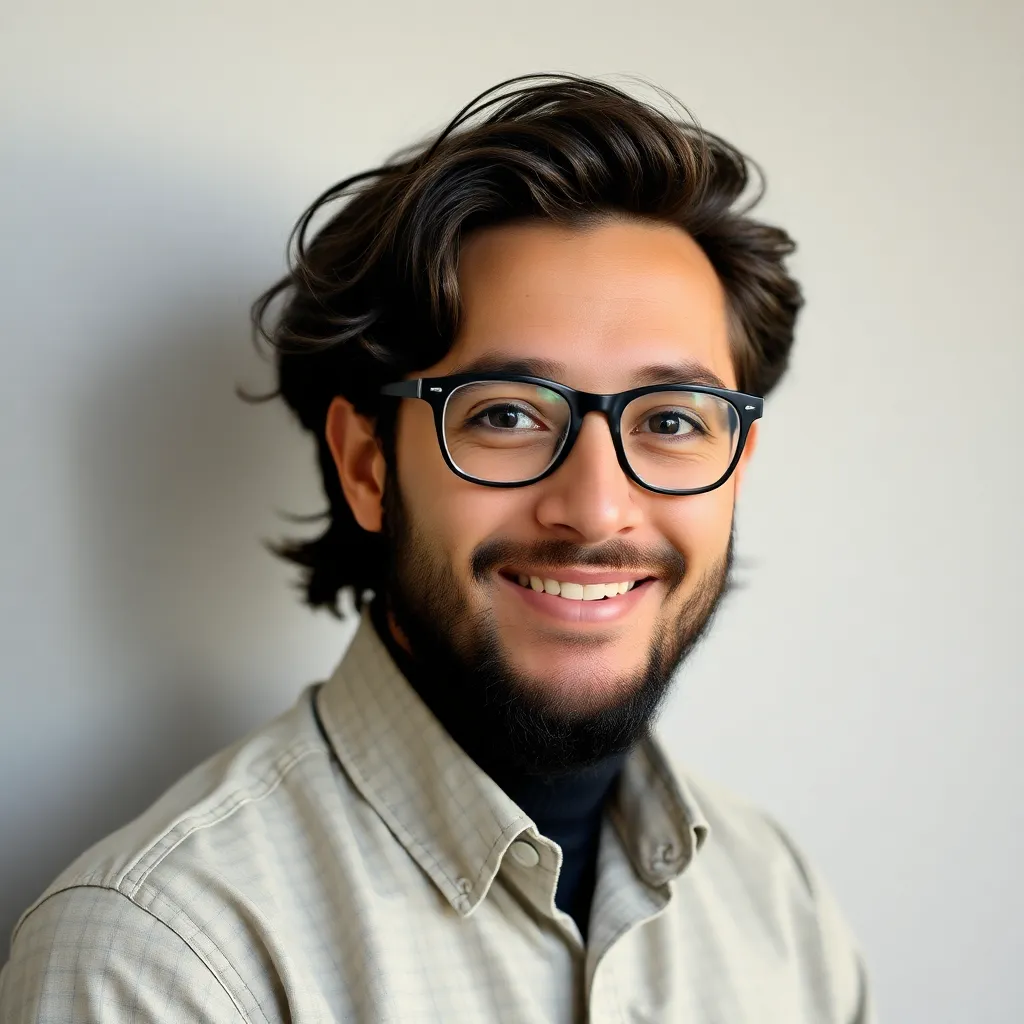
Juapaving
Apr 03, 2025 · 7 min read
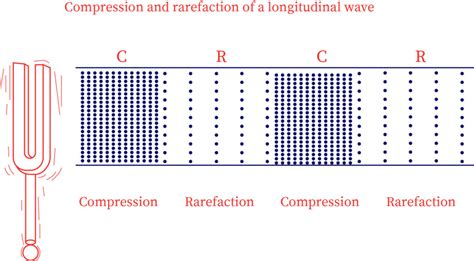
Table of Contents
Compressions and Rarefactions: Characteristics of Longitudinal Waves
Compressions and rarefactions are fundamental characteristics that define longitudinal waves. Understanding these concepts is crucial for grasping various phenomena in physics, from the propagation of sound to the behavior of seismic waves. This article will delve deep into the nature of compressions and rarefactions, exploring their relationship to longitudinal waves, providing real-world examples, and clarifying common misconceptions.
What are Compressions and Rarefactions?
Imagine a slinky toy. If you push one end, you create a region where the coils are tightly packed together. This region of increased density is a compression. Conversely, if you pull on the slinky, you create a region where the coils are spread out, a region of decreased density called a rarefaction.
These compressions and rarefactions are not static; they travel along the slinky as a wave. This type of wave, where the particle displacement is parallel to the direction of wave propagation, is called a longitudinal wave. In contrast to transverse waves, where particle displacement is perpendicular to the wave propagation (like ripples in water), longitudinal waves involve the rhythmic compression and rarefaction of the medium.
The Mechanism of Wave Propagation
The propagation of a longitudinal wave relies on the interaction between particles within the medium. When a compression is created, the particles in that region are pushed closer together. This increased pressure causes these particles to push on their neighboring particles, transmitting the compression further down the line. Similarly, the particles in a rarefaction region are further apart, creating a region of lower pressure. This pressure difference causes the particles to move slightly apart, propagating the rarefaction.
This process continues, creating a continuous sequence of compressions and rarefactions that propagate through the medium as a wave. The speed of this propagation depends on the properties of the medium, such as its elasticity and density. A stiffer, denser medium will generally transmit longitudinal waves faster than a less stiff, less dense medium.
Understanding the Relationship with Pressure and Density
The compressions and rarefactions in a longitudinal wave are directly related to the pressure and density variations within the medium. Compressions are characterized by:
- Increased pressure: The particles are closer together, leading to a higher pressure in that region.
- Increased density: The concentration of particles is higher, resulting in increased density.
Rarefactions, on the other hand, are characterized by:
- Decreased pressure: The particles are further apart, leading to a lower pressure in that region.
- Decreased density: The concentration of particles is lower, resulting in decreased density.
These pressure and density variations are essential for the transmission of energy through the medium. The wave's energy is carried by the oscillating pressure and density variations, not by the bulk movement of the medium itself. Particles in the medium oscillate around their equilibrium positions, transferring energy along the wave without significant net displacement.
Real-World Examples of Compressions and Rarefactions
Longitudinal waves, characterized by their compressions and rarefactions, are ubiquitous in the natural world and have significant technological applications. Here are some key examples:
1. Sound Waves:
Sound waves are perhaps the most familiar example of longitudinal waves. When an object vibrates, it creates alternating compressions and rarefactions in the surrounding air. These compressions and rarefactions travel through the air as sound waves, eventually reaching our ears where they are interpreted as sound. The frequency of these compressions and rarefactions determines the pitch of the sound, while their amplitude determines the loudness.
Different media transmit sound waves at different speeds. Sound travels faster in solids than in liquids, and faster in liquids than in gases. This difference stems from the varying densities and elasticity of these different media. The closer the particles are in a medium, the faster they can transmit the compression and rarefaction.
2. Seismic Waves:
Seismic waves are longitudinal waves generated during earthquakes. These waves travel through the Earth's interior, causing ground shaking. The primary waves (P-waves) are longitudinal waves that travel faster than secondary waves (S-waves), which are transverse waves. The arrival times of P-waves and S-waves at seismograph stations are used to determine the location and magnitude of earthquakes.
The analysis of seismic waves provides crucial insights into the Earth's internal structure. The way these waves travel through different layers of the Earth, including variations in speed and reflection patterns, allows geophysicists to map the Earth's layers, identifying boundaries between different materials and determining their properties.
3. Ultrasound:
Ultrasound technology utilizes high-frequency sound waves to create images of internal organs and structures. These sound waves are longitudinal waves that are reflected by different tissues and organs within the body. The reflected waves are detected and used to create images, which are essential for medical diagnosis and treatment.
The use of ultrasound is non-invasive and safe, making it a valuable tool in various medical applications, including prenatal imaging, detecting tumors, and guiding biopsies. The ability of ultrasound to penetrate tissues and differentiate between different types of tissue based on the reflection and scattering of the sound waves is a testament to the power of understanding longitudinal waves.
4. Ultrasonic Cleaning:
Ultrasonic cleaning utilizes high-frequency sound waves to clean delicate objects. The compressions and rarefactions created by these sound waves cause cavitation – the formation and collapse of tiny bubbles in the cleaning solution. The collapsing bubbles generate powerful localized pressure changes that dislodge dirt and debris from the objects being cleaned.
This technique is effective for cleaning intricate parts, jewelry, and other delicate objects that would be damaged by more abrasive cleaning methods. The effectiveness of ultrasonic cleaning hinges on the precise control of the frequency and intensity of the sound waves, utilizing the power of compressions and rarefactions to achieve a superior level of cleanliness.
Distinguishing Longitudinal Waves from Transverse Waves
It's crucial to differentiate between longitudinal and transverse waves. While both are types of mechanical waves requiring a medium for propagation, their particle motion differs significantly:
- Longitudinal Waves: Particle displacement is parallel to the direction of wave propagation. They are characterized by compressions and rarefactions.
- Transverse Waves: Particle displacement is perpendicular to the direction of wave propagation. They are characterized by crests (high points) and troughs (low points).
Understanding this distinction is key to interpreting the behavior of various waves in different contexts. For example, sound waves are longitudinal, while light waves are transverse (electromagnetic waves, not requiring a medium). Seismic waves include both P-waves (longitudinal) and S-waves (transverse).
Applications and Significance
The understanding of compressions and rarefactions, as the defining characteristics of longitudinal waves, has profound implications across various scientific and technological fields. From medical imaging to earthquake prediction, from material testing to musical instrument design, the principles governing the propagation and interaction of longitudinal waves are essential.
The development of new technologies continues to rely on a deeper understanding of wave behavior. Advances in materials science, for instance, often involve investigating how different materials respond to longitudinal waves, leading to the development of new materials with specific properties. Similarly, developments in medical imaging and therapeutic techniques continually refine the use of longitudinal waves for improved diagnostic capabilities and treatment options.
Furthermore, a thorough understanding of compressions and rarefactions enables the design of improved acoustic devices, from speakers and microphones to sonar systems. Precise control over the generation and manipulation of these waves is critical for achieving optimal performance and efficiency in a wide array of applications.
Common Misconceptions
Several common misconceptions surround compressions and rarefactions:
-
Compressions and rarefactions are not the particles themselves: The particles in the medium oscillate around their equilibrium positions; they are not permanently displaced by the wave. The compression and rarefaction represent regions of higher and lower particle density, respectively.
-
The medium itself does not travel with the wave: The wave propagates through the medium, transferring energy, but the medium itself does not travel along with the wave. The particles oscillate locally, transferring energy through their interactions.
-
Compressions and rarefactions are not limited to air: While sound waves often serve as an example, compressions and rarefactions occur in various media, including solids, liquids, and gases. The properties of the medium affect the speed of wave propagation.
Conclusion
Compressions and rarefactions are integral components of longitudinal waves, playing a pivotal role in diverse phenomena across various scientific disciplines and technological applications. A thorough grasp of these concepts is fundamental to comprehending wave behavior, enabling the development of innovative technologies and the advancement of scientific knowledge. From understanding the propagation of sound to harnessing the power of seismic waves and ultrasound, the study of compressions and rarefactions opens up a world of possibilities. Continuous research and innovation in this area promise to further our understanding of the universe and lead to the development of groundbreaking technologies in the future.
Latest Posts
Latest Posts
-
How Many Liters Has In Half Gallon
Apr 03, 2025
-
What Is 20 Percent Of 150
Apr 03, 2025
-
Electrical Current Will Pass Easily Through
Apr 03, 2025
-
Which Of The Following Are Rational Numbers
Apr 03, 2025
-
Five Letter Words With Ie In Them
Apr 03, 2025
Related Post
Thank you for visiting our website which covers about Compressions And Rarefactions Are Characteristics Of . We hope the information provided has been useful to you. Feel free to contact us if you have any questions or need further assistance. See you next time and don't miss to bookmark.