Where Glucose Gets Broken Into Pyruvate In The Cell
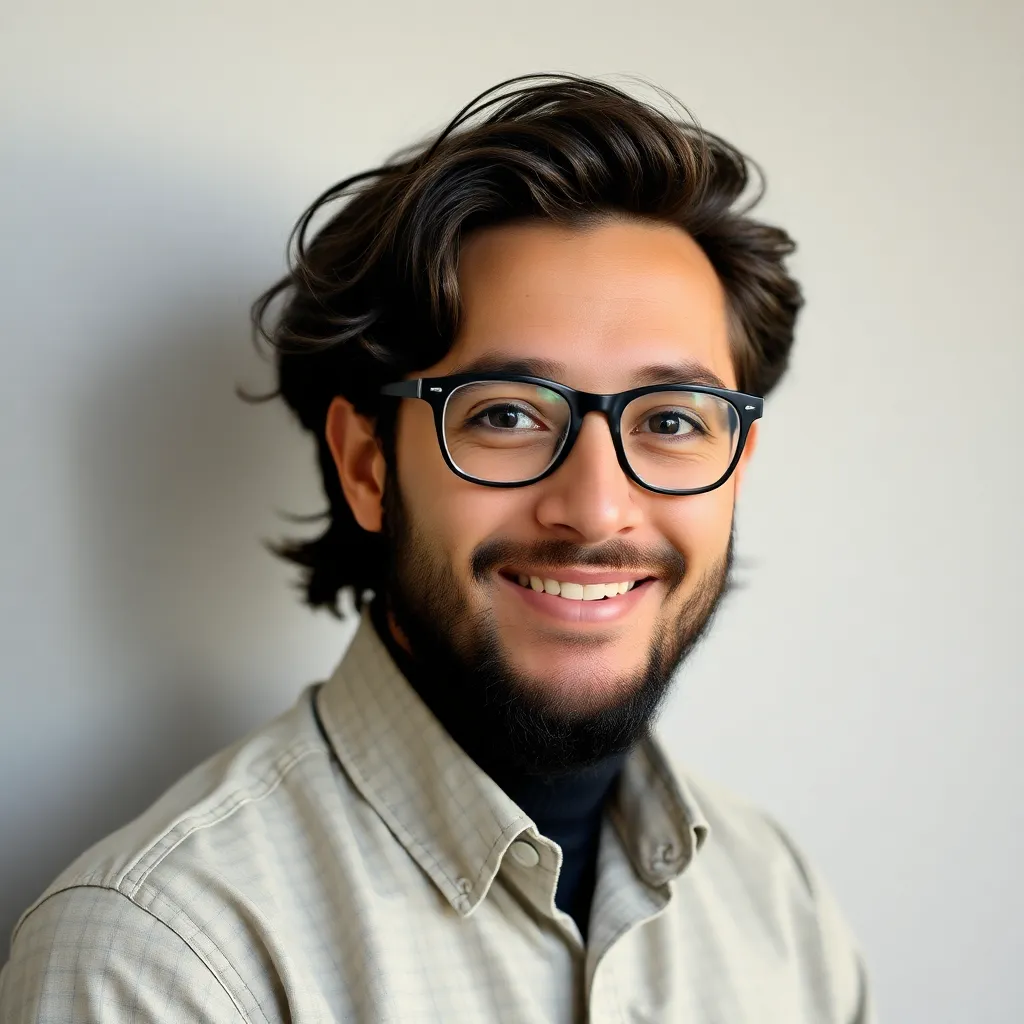
Juapaving
Apr 01, 2025 · 6 min read
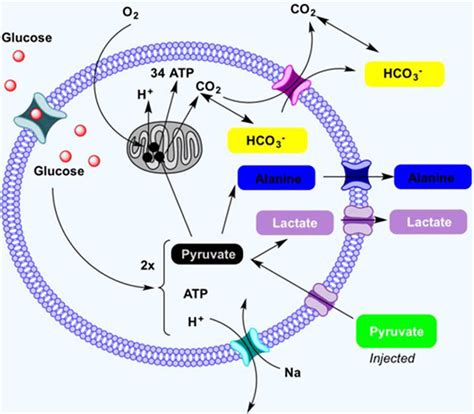
Table of Contents
Where Glucose Gets Broken Down into Pyruvate in the Cell: A Deep Dive into Glycolysis
Glycolysis, the metabolic pathway responsible for breaking down glucose into pyruvate, is a fundamental process in nearly all living organisms. Understanding where and how this crucial reaction occurs is essential to grasping cellular energy production and its implications for various biological processes. This article will delve into the intricate details of glycolysis, exploring its location within the cell, the specific steps involved, and the regulation mechanisms that control this vital pathway.
The Cellular Location of Glycolysis: The Cytoplasm
Unlike many other metabolic pathways that occur within specific organelles, glycolysis takes place entirely in the cytoplasm, the gel-like substance that fills the cell and surrounds the organelles. This cytoplasmic location is crucial because it allows for a rapid and efficient breakdown of glucose without the need for transport across multiple membranes. The enzymes responsible for each step of glycolysis are freely dissolved in the cytoplasm or loosely associated with the cytoskeleton, ensuring proximity and facilitating the rapid transfer of intermediates between enzymatic reactions.
Why the Cytoplasm? Evolutionary Advantages and Efficiency
The cytoplasmic location of glycolysis likely reflects its evolutionary origins as an ancient metabolic pathway. Before the evolution of complex organelles like mitochondria, all cellular metabolism occurred in the cytoplasm. This early reliance on cytoplasmic processes explains the continued localization of glycolysis in this cellular compartment, even in eukaryotic cells with highly developed organelles.
The cytoplasmic location also provides several practical advantages. The substrates and enzymes are readily available, reducing the energy costs associated with transporting molecules across membranes. The proximity of enzymes allows for efficient channeling of metabolic intermediates, minimizing diffusion losses and accelerating the overall rate of glucose breakdown.
The Ten Steps of Glycolysis: A Detailed Breakdown
Glycolysis is a ten-step pathway that can be broadly divided into two phases: the energy-investment phase and the energy-payoff phase.
Phase 1: The Energy-Investment Phase (Steps 1-5)
This phase requires an initial investment of ATP to phosphorylate glucose, making it more reactive and preparing it for subsequent cleavage.
-
Hexokinase: This enzyme catalyzes the phosphorylation of glucose to glucose-6-phosphate (G6P), utilizing one molecule of ATP. This step is crucial for trapping glucose within the cell, as G6P cannot readily cross the cell membrane. The regulation of hexokinase is pivotal in controlling the rate of glycolysis.
-
Phosphoglucose Isomerase: G6P is isomerized to fructose-6-phosphate (F6P), converting an aldose (glucose) to a ketose (fructose). This isomerization is essential for the subsequent cleavage reaction.
-
Phosphofructokinase (PFK): This is the rate-limiting enzyme of glycolysis. PFK catalyzes the phosphorylation of F6P to fructose-1,6-bisphosphate (F1,6BP), using another molecule of ATP. This step is highly regulated, responding to cellular energy levels and other metabolic signals.
-
Aldolase: F1,6BP is cleaved into two three-carbon molecules: glyceraldehyde-3-phosphate (G3P) and dihydroxyacetone phosphate (DHAP).
-
Triose Phosphate Isomerase: DHAP is isomerized to G3P. This step ensures that all the carbon atoms from glucose are channeled into the subsequent energy-generating steps.
Phase 2: The Energy-Payoff Phase (Steps 6-10)
This phase generates ATP and NADH, yielding a net energy gain for the cell. Note that each step in this phase occurs twice for each initial glucose molecule because of the cleavage into two three-carbon molecules in step 4.
-
Glyceraldehyde-3-Phosphate Dehydrogenase (GAPDH): G3P is oxidized and phosphorylated, yielding 1,3-bisphosphoglycerate (1,3BPG) and reducing NAD+ to NADH. This oxidation step is coupled to the formation of a high-energy phosphate bond.
-
Phosphoglycerate Kinase: 1,3BPG transfers a high-energy phosphate group to ADP, generating ATP and forming 3-phosphoglycerate (3PG). This is the first substrate-level phosphorylation step, generating ATP directly without the need for an electron transport chain.
-
Phosphoglycerate Mutase: 3PG is isomerized to 2-phosphoglycerate (2PG). This isomerization is necessary for the next step.
-
Enolase: 2PG undergoes dehydration, forming phosphoenolpyruvate (PEP), a high-energy phosphate compound.
-
Pyruvate Kinase: PEP transfers its high-energy phosphate group to ADP, generating another molecule of ATP and forming pyruvate. This is the second substrate-level phosphorylation.
The Fate of Pyruvate: Beyond Glycolysis
The pyruvate generated during glycolysis has several possible fates, depending on the cellular environment and the organism's metabolic needs.
-
Aerobic Conditions (Presence of Oxygen): Under aerobic conditions, pyruvate enters the mitochondria and is further oxidized in the citric acid cycle (also known as the Krebs cycle or TCA cycle). This process generates more ATP, NADH, and FADH2, which are then used in oxidative phosphorylation to generate a significant amount of ATP via the electron transport chain.
-
Anaerobic Conditions (Absence of Oxygen): In the absence of oxygen, pyruvate undergoes fermentation. In humans and other animals, this results in the formation of lactate. In yeast and some bacteria, pyruvate is converted to ethanol and carbon dioxide through alcoholic fermentation. These fermentation pathways regenerate NAD+ from NADH, allowing glycolysis to continue even without oxygen.
Regulation of Glycolysis: A Symphony of Control
Glycolysis is a tightly regulated pathway, ensuring that glucose is metabolized efficiently and appropriately in response to the cell's energy demands. Several key enzymes are subject to allosteric regulation, meaning their activity is modulated by the binding of small molecules.
-
Hexokinase: Is inhibited by its product, G6P, providing feedback inhibition.
-
Phosphofructokinase (PFK): This is the primary regulatory enzyme of glycolysis. It is activated by AMP and ADP (indicating low energy levels) and inhibited by ATP and citrate (indicating high energy levels). It is also inhibited by high levels of hydrogen ions, reflecting the cellular response to acidosis.
-
Pyruvate Kinase: This enzyme is also regulated by energy levels, being activated by fructose-1,6-bisphosphate (a feed-forward mechanism) and inhibited by ATP and acetyl-CoA (signals of high energy status).
The integration of these regulatory mechanisms ensures that glycolysis operates at the appropriate rate to meet the cell's energy needs while avoiding wasteful overproduction of metabolites.
Clinical Significance and Diseases Related to Glycolytic Dysfunction
Disruptions in glycolysis can lead to serious health consequences. Several inherited metabolic disorders affect enzymes involved in glycolysis, resulting in impaired glucose metabolism and a range of symptoms. Examples include:
-
Pyruvate kinase deficiency: This leads to hemolytic anemia due to reduced ATP production in red blood cells.
-
Phosphofructokinase deficiency: Causes muscle weakness and cramps.
-
Deficiencies in other glycolytic enzymes: Can lead to a range of symptoms, depending on the specific enzyme and the severity of the deficiency.
Furthermore, cancer cells often exhibit altered glycolytic activity, exhibiting a phenomenon known as the Warburg effect, where they preferentially use glycolysis even in the presence of oxygen. This shift in metabolism provides cancer cells with a growth advantage, making glycolysis a crucial target for cancer therapy.
Conclusion: A Fundamental Pathway with Far-Reaching Implications
Glycolysis, the cytoplasmic pathway that breaks down glucose to pyruvate, is a fundamental metabolic process essential for cellular energy production. Its location in the cytoplasm, the intricate steps involved, and the complex regulatory mechanisms all contribute to its critical role in maintaining cellular homeostasis. A deep understanding of glycolysis and its regulation is not only important for basic biological research but also holds significant clinical implications, particularly in the understanding and treatment of metabolic disorders and cancer. Further research continues to illuminate the fascinating details of this ubiquitous and vital pathway.
Latest Posts
Latest Posts
-
Does A Gas Have A Definite Volume
Apr 02, 2025
-
Which Base Is Found Only In Rna
Apr 02, 2025
-
Difference Between Meiosis 1 And Meiosis 2
Apr 02, 2025
-
Equation Of Circle In Parametric Form
Apr 02, 2025
-
How To Find The Complement Of An Angle
Apr 02, 2025
Related Post
Thank you for visiting our website which covers about Where Glucose Gets Broken Into Pyruvate In The Cell . We hope the information provided has been useful to you. Feel free to contact us if you have any questions or need further assistance. See you next time and don't miss to bookmark.