What Bond Holds The Nitrogen Bases Together
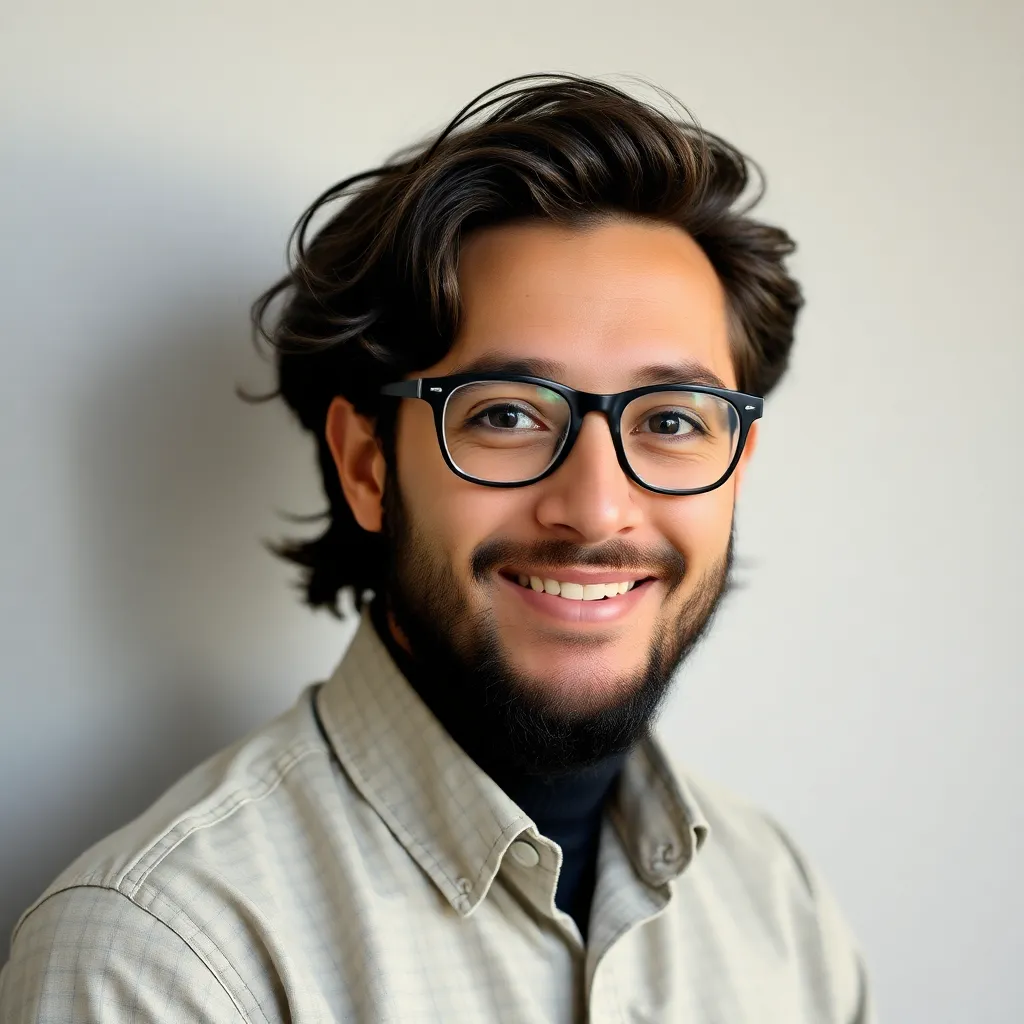
Juapaving
Apr 06, 2025 · 6 min read
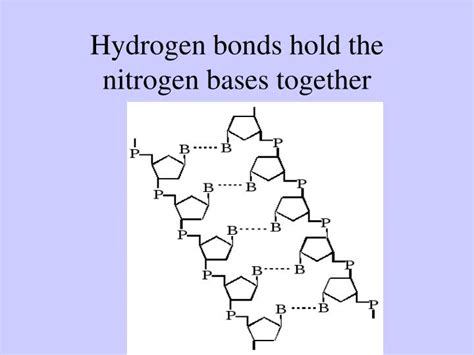
Table of Contents
What Bond Holds the Nitrogenous Bases Together? The Fascinating World of Hydrogen Bonds in DNA
The very essence of life, as we know it, hinges on the precise pairing of nitrogenous bases within the DNA double helix. This pairing, the foundation of heredity and genetic information transfer, isn't achieved through some mystical force, but through the elegant and relatively weak chemical bond known as the hydrogen bond. Understanding the nature and strength of these bonds is crucial to grasping the mechanics of DNA replication, transcription, and ultimately, life itself.
Decoding the DNA Double Helix: A Molecular Marvel
Deoxyribonucleic acid (DNA) is the blueprint of life, a complex molecule composed of two intertwined strands forming the iconic double helix. Each strand is a chain of nucleotides, which are further composed of three key components:
- A deoxyribose sugar: A five-carbon sugar molecule that forms the backbone of the DNA strand.
- A phosphate group: Linking the sugar molecules together to create the sugar-phosphate backbone.
- A nitrogenous base: One of four key molecules – adenine (A), guanine (G), cytosine (C), and thymine (T) – that project inwards from the sugar-phosphate backbone, forming the "rungs" of the DNA ladder.
These nitrogenous bases are aromatic heterocyclic organic compounds, meaning they contain rings of carbon and nitrogen atoms and exhibit special properties due to their conjugated π-electron systems. It’s the interaction between these bases that holds the two DNA strands together.
The Hydrogen Bond: A Weak but Vital Link
The key to understanding the pairing of nitrogenous bases lies in the hydrogen bond, a type of dipole-dipole interaction. This is a special kind of electrostatic attraction, weaker than covalent bonds but stronger than van der Waals forces. A hydrogen bond occurs when a hydrogen atom covalently bonded to a highly electronegative atom (like oxygen or nitrogen) is also weakly attracted to another electronegative atom in a nearby molecule or part of the same molecule. In the case of DNA, this electronegative atom is typically oxygen or nitrogen within another base.
Understanding Electronegativity and Polarity
The concept of electronegativity plays a crucial role here. Electronegativity is the ability of an atom to attract electrons within a covalent bond. Oxygen and nitrogen are highly electronegative atoms; they strongly attract electrons towards themselves, creating a partial negative charge (δ-) on the oxygen or nitrogen atom and a partial positive charge (δ+) on the hydrogen atom. This creates a dipole moment – a separation of charge within the molecule.
It's this partial positive charge on the hydrogen (δ+) that is attracted to the partial negative charge (δ-) on the electronegative atom of the adjacent base. This attraction is the hydrogen bond.
Base Pairing Specificity: Chargaff's Rules and Watson-Crick Model
Erwin Chargaff's rules, established in the mid-20th century, demonstrated a consistent ratio of specific bases within DNA: the amount of adenine (A) always equaled the amount of thymine (T), and the amount of guanine (G) always equaled the amount of cytosine (C). This observation provided crucial evidence for the later development of the Watson-Crick model of DNA structure.
The Watson-Crick model explained Chargaff's rules by proposing specific base pairing:
- Adenine (A) always pairs with Thymine (T)
- Guanine (G) always pairs with Cytosine (C)
This specific pairing is dictated by the geometry of the bases and the number and position of hydrogen bonds that can be formed.
Adenine-Thymine (A-T) Pairing: Two Hydrogen Bonds
The A-T base pair is held together by two hydrogen bonds. One hydrogen bond forms between the amino group (-NH2) of adenine and the carbonyl group (=O) of thymine. The second hydrogen bond forms between the nitrogen atom of adenine and another nitrogen atom of thymine. These two hydrogen bonds work in concert to stabilize the A-T base pair within the DNA double helix.
Guanine-Cytosine (G-C) Pairing: Three Hydrogen Bonds
The G-C base pair is held together by three hydrogen bonds, making it slightly stronger than the A-T base pair. The three hydrogen bonds are distributed among the amino and carbonyl groups of guanine and cytosine, creating a more robust interaction. The stronger G-C base pair contributes to the overall stability of DNA, with regions rich in G-C having a higher melting point (requiring higher temperatures to separate the strands).
The Significance of Hydrogen Bonds in DNA Function
The relatively weak nature of hydrogen bonds is crucial for DNA's function. The fact that they are weaker than covalent bonds allows for:
- Easy strand separation: The relatively weak hydrogen bonds can be easily broken and reformed, enabling DNA replication and transcription. Enzymes like DNA helicases unwind the double helix, breaking the hydrogen bonds between the base pairs, allowing access to the genetic information encoded within the sequence.
- Specific base pairing: The precise geometry and number of hydrogen bonds dictate the specific pairing between A-T and G-C, ensuring the fidelity of DNA replication and maintaining the integrity of the genetic code. Mismatched base pairs would have either fewer or more hydrogen bonds, making them less stable and more likely to be corrected by DNA repair mechanisms.
- DNA flexibility: The flexibility afforded by hydrogen bonds allows the DNA molecule to bend and twist, interacting with proteins and other molecules involved in DNA replication, transcription, and repair.
Beyond Hydrogen Bonds: Other Intermolecular Forces in DNA Stability
While hydrogen bonds are the primary force responsible for base pairing, other intermolecular forces contribute to the overall stability of the DNA double helix:
- Hydrophobic interactions: The bases are relatively hydrophobic (water-repelling), and their stacking within the DNA helix minimizes their contact with water, contributing to stability. This stacking interaction is often called base-stacking and is significant in stabilizing the helix.
- Van der Waals forces: These are weak, short-range attractive forces between molecules. They contribute to the overall stability, especially between stacked base pairs.
- Electrostatic interactions: The negatively charged phosphate groups in the DNA backbone repel each other, contributing to the overall structure of the double helix and influencing DNA's interactions with proteins and ions. These negative charges are often balanced by the presence of positively charged ions (like Mg2+) in the cellular environment.
Conclusion: The Hydrogen Bond - A Masterpiece of Molecular Engineering
The hydrogen bond, while individually weak, is the linchpin that holds together the building blocks of life. Its role in mediating the specific base pairing in DNA underpins the intricate processes of replication, transcription, and translation, ensuring the faithful transmission of genetic information across generations. The delicate balance of strength and weakness, combined with other intermolecular forces, makes the hydrogen bond a remarkable example of molecular engineering – a testament to the elegant design of life itself. Future research continues to explore the nuances of hydrogen bonding within DNA, furthering our understanding of this fundamental biological process and paving the way for potential advancements in fields such as gene editing and DNA sequencing technologies.
Latest Posts
Latest Posts
-
How Do You Analyze A Graph
Apr 08, 2025
-
The Oxygen Produced In Photosynthesis Comes From What Molecule
Apr 08, 2025
-
Benedicts Test Shows The Presence Of
Apr 08, 2025
-
Choose The Correct Answer From The Given Options
Apr 08, 2025
-
What Is 62 5 As A Fraction
Apr 08, 2025
Related Post
Thank you for visiting our website which covers about What Bond Holds The Nitrogen Bases Together . We hope the information provided has been useful to you. Feel free to contact us if you have any questions or need further assistance. See you next time and don't miss to bookmark.