The Genetic Material Is Duplicated During
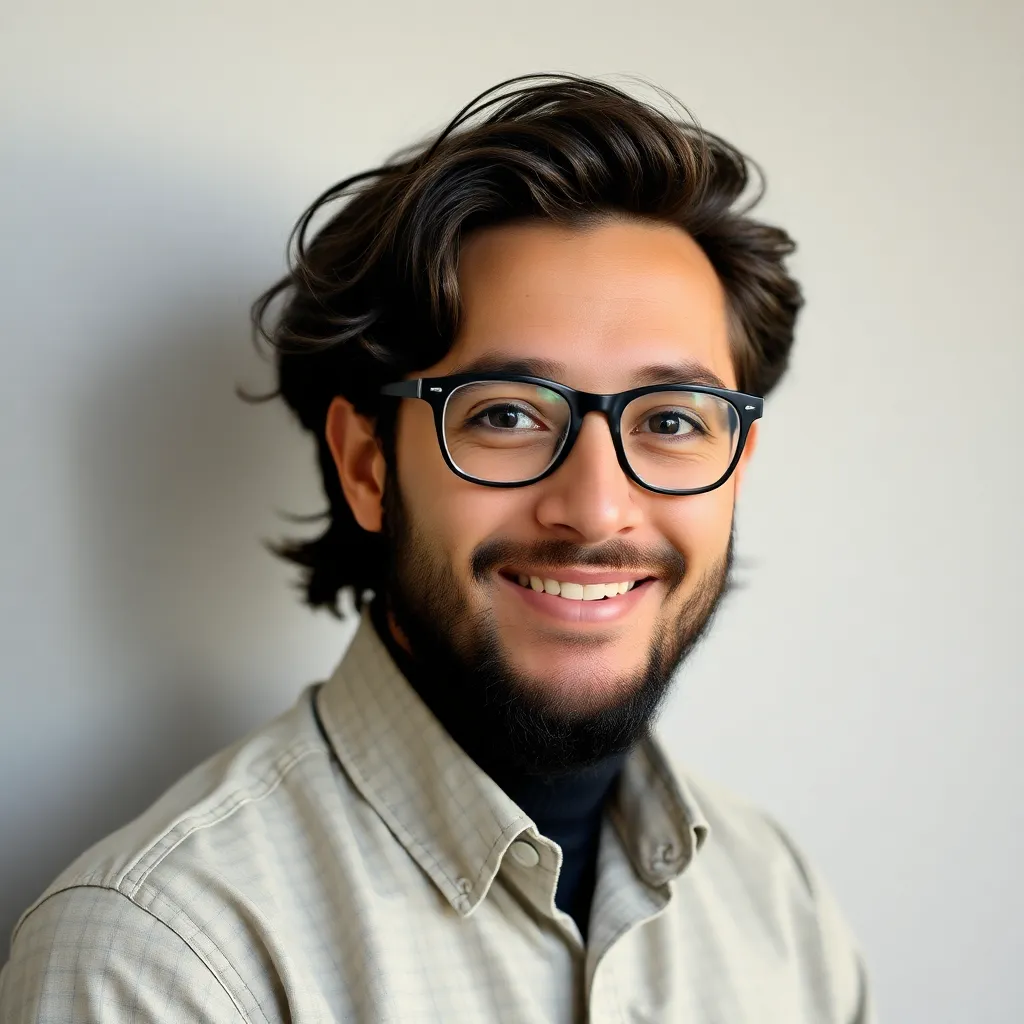
Juapaving
May 10, 2025 · 6 min read
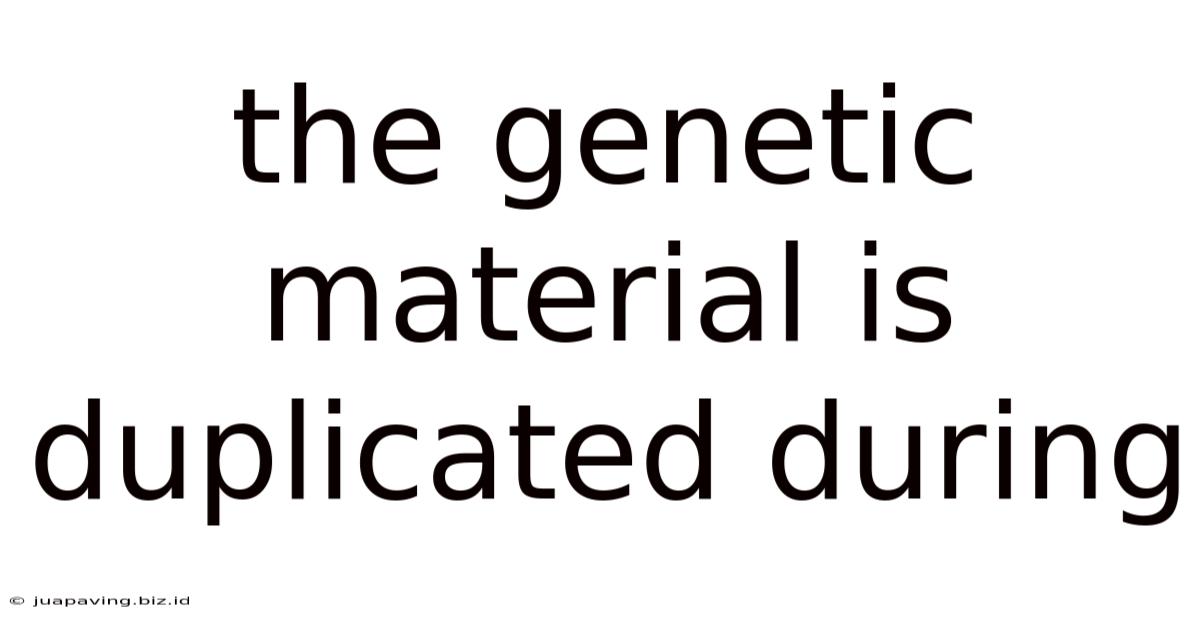
Table of Contents
The Genetic Material is Duplicated During: DNA Replication – A Deep Dive
DNA replication is a fundamental process in all living organisms, essential for cell growth, repair, and reproduction. It's the precise duplication of the entire genome, ensuring that each daughter cell receives an identical copy of the genetic material. Understanding this intricate process is crucial to comprehending the mechanisms of heredity, evolution, and various diseases. This article delves into the fascinating world of DNA replication, exploring its phases, key enzymes, regulatory mechanisms, and its importance in maintaining genomic integrity.
The Central Dogma and the Necessity of Replication
Before diving into the specifics, let's briefly revisit the central dogma of molecular biology: DNA makes RNA makes protein. This elegant framework highlights the flow of genetic information. However, for this flow to continue across generations, the DNA itself must be accurately copied. DNA replication ensures that genetic information is passed faithfully from one cell to its progeny. Without it, cells would be unable to divide, and life as we know it wouldn't exist.
The Semi-Conservative Nature of DNA Replication
A cornerstone of our understanding of DNA replication is its semi-conservative nature. This means that each new DNA molecule consists of one original (parental) strand and one newly synthesized strand. This discovery, made by Meselson and Stahl, elegantly explained how the genetic material is faithfully copied without losing information. This semi-conservative mechanism ensures accuracy and reduces the chances of errors during replication.
The Players: Key Enzymes and Proteins Involved
DNA replication is a highly coordinated process involving a complex orchestra of enzymes and proteins, each playing a crucial role in the fidelity and efficiency of the process. Let's examine some of the key players:
1. DNA Helicase: Unwinding the Double Helix
DNA helicase is like a molecular zipper, unwinding the double helix at the origin of replication, creating a replication fork – the Y-shaped region where the DNA strands separate. This unwinding creates single-stranded DNA templates for replication. The helicase's activity is crucial because it overcomes the strong hydrogen bonds holding the two strands together.
2. Single-Strand Binding Proteins (SSBs): Stabilizing the Templates
Once the double helix is unwound, the single-stranded DNA templates are vulnerable to degradation or re-annealing. SSBs bind to the single-stranded DNA, preventing these undesirable events and keeping the templates stable for the upcoming polymerase action. They act as protective chaperones, ensuring the integrity of the single-stranded DNA.
3. DNA Primase: Synthesizing RNA Primers
DNA polymerase, the enzyme responsible for synthesizing new DNA strands, cannot initiate synthesis de novo. It requires a pre-existing 3'-OH group to add nucleotides to. This is where DNA primase comes in. DNA primase synthesizes short RNA primers, providing the necessary 3'-OH group for DNA polymerase to begin its work. These RNA primers are later removed and replaced with DNA.
4. DNA Polymerase: Building the New Strands
DNA polymerase is the central enzyme in DNA replication. There are several types of DNA polymerases, each with specific roles. The main polymerase responsible for the bulk of DNA synthesis is usually DNA polymerase III in prokaryotes and several different polymerases in eukaryotes. These enzymes add nucleotides to the 3' end of the growing strand, following the base-pairing rules (A with T, and G with C). This process is incredibly accurate, with an error rate of only about one mistake per billion base pairs.
5. DNA Ligase: Sealing the Gaps
As the new DNA strands are synthesized, fragments called Okazaki fragments are created on the lagging strand. These fragments are joined together by DNA ligase, an enzyme that catalyzes the formation of phosphodiester bonds between the adjacent fragments, creating a continuous strand.
6. Topoisomerases: Relieving Torsional Stress
As the DNA unwinds, torsional stress builds up ahead of the replication fork. Topoisomerases are enzymes that alleviate this stress by cutting and rejoining DNA strands. They prevent the DNA from becoming supercoiled and tangled, ensuring smooth and efficient replication.
The Leading and Lagging Strands: A Tale of Two Strands
Because DNA polymerase can only add nucleotides to the 3' end, the synthesis of the two new strands proceeds differently. The leading strand is synthesized continuously in the 5' to 3' direction, following the replication fork. The lagging strand, on the other hand, is synthesized discontinuously in short fragments called Okazaki fragments, each requiring a separate RNA primer. This difference reflects the antiparallel nature of the DNA double helix.
Proofreading and Repair Mechanisms: Maintaining Fidelity
The fidelity of DNA replication is paramount. Despite the high accuracy of DNA polymerase, errors can occur. Fortunately, cells possess sophisticated proofreading and repair mechanisms to correct these mistakes. DNA polymerase itself has a proofreading function, removing incorrectly incorporated nucleotides. In addition, various repair pathways, such as mismatch repair and nucleotide excision repair, further enhance the accuracy of replication and maintain genomic stability. These mechanisms are crucial for preventing mutations that can lead to diseases and genomic instability.
Replication in Eukaryotes vs. Prokaryotes: Similarities and Differences
While the basic principles of DNA replication are conserved across all life forms, there are some key differences between prokaryotic and eukaryotic replication. Prokaryotic replication is simpler, typically involving a single origin of replication and a smaller genome. Eukaryotic replication is significantly more complex, involving multiple origins of replication, a larger genome, and more intricate regulatory mechanisms to ensure timely and coordinated replication of the entire genome. The presence of nucleosomes and other chromatin structures in eukaryotes also adds complexity to the process.
Telomeres and Telomerase: Protecting the Ends of Chromosomes
Linear eukaryotic chromosomes pose a unique challenge to DNA replication. The lagging strand synthesis cannot completely replicate the very end of the chromosome, leading to a gradual shortening of the telomeres – repetitive DNA sequences at the chromosome ends. This shortening can have detrimental effects on cell function and lifespan. Telomerase, a specialized reverse transcriptase, helps counteract telomere shortening by adding telomeric repeats to the chromosome ends, preserving genomic integrity.
Regulation of DNA Replication: Timing and Control
DNA replication is not a continuous process; it's tightly regulated to ensure that the genome is duplicated only once per cell cycle. Various checkpoints and regulatory mechanisms control the initiation, elongation, and termination of replication. These mechanisms ensure that replication is coordinated with other cellular processes and that errors are minimized. Dysregulation of these mechanisms can lead to genomic instability and diseases such as cancer.
The Significance of DNA Replication in Health and Disease
Faithful DNA replication is essential for maintaining genomic stability and cellular health. Errors in DNA replication can lead to mutations, which can cause a wide range of diseases, including cancer, genetic disorders, and aging-related diseases. Understanding the mechanisms of DNA replication and its regulation is therefore crucial for developing therapies for these diseases.
Future Directions in DNA Replication Research
Research in DNA replication continues to be a vibrant field, with ongoing efforts to unravel the intricacies of this essential process. Future research will likely focus on:
- Understanding the precise mechanisms of replication fork stalling and restart: These events are crucial for maintaining replication fidelity in the face of challenges such as DNA damage.
- Investigating the roles of specific proteins and complexes in regulating replication: This research is essential for understanding how replication is coordinated with other cellular processes.
- Developing new therapeutic strategies targeting DNA replication processes: This research holds immense promise for treating diseases caused by replication errors.
In conclusion, DNA replication is a marvel of biological engineering, a highly precise and intricately regulated process that underpins the continuity of life. Understanding this fundamental process is crucial for advancing our knowledge of heredity, evolution, and disease. The ongoing research in this field promises exciting discoveries that will reshape our understanding of life itself.
Latest Posts
Latest Posts
-
Which Of The Following Is An Example Of Physical Capital
May 10, 2025
-
1 1 2 On A Number Line
May 10, 2025
-
How Are Energy And Work Related
May 10, 2025
-
Contains Dna And Controls Cell Activities
May 10, 2025
-
Six And Half Inches In Cm
May 10, 2025
Related Post
Thank you for visiting our website which covers about The Genetic Material Is Duplicated During . We hope the information provided has been useful to you. Feel free to contact us if you have any questions or need further assistance. See you next time and don't miss to bookmark.