How Are Energy And Work Related
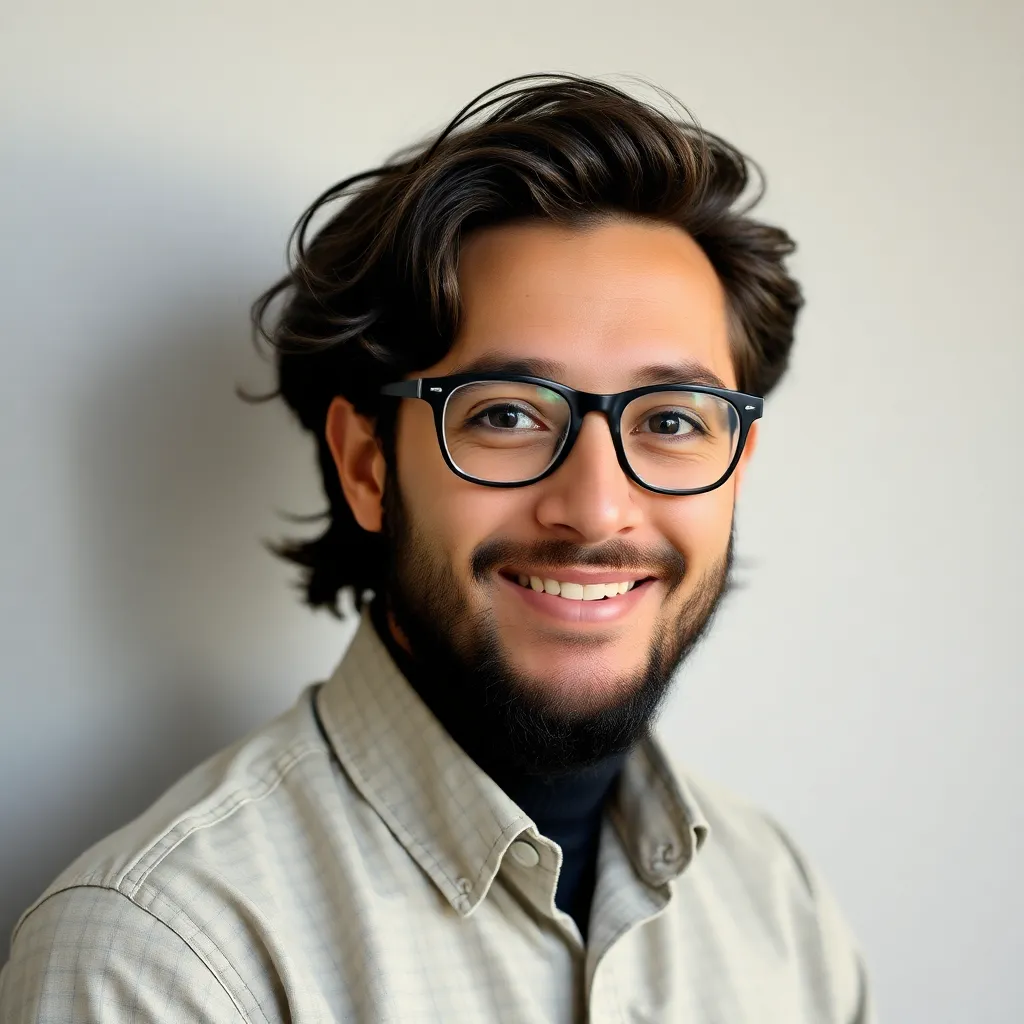
Juapaving
May 10, 2025 · 7 min read
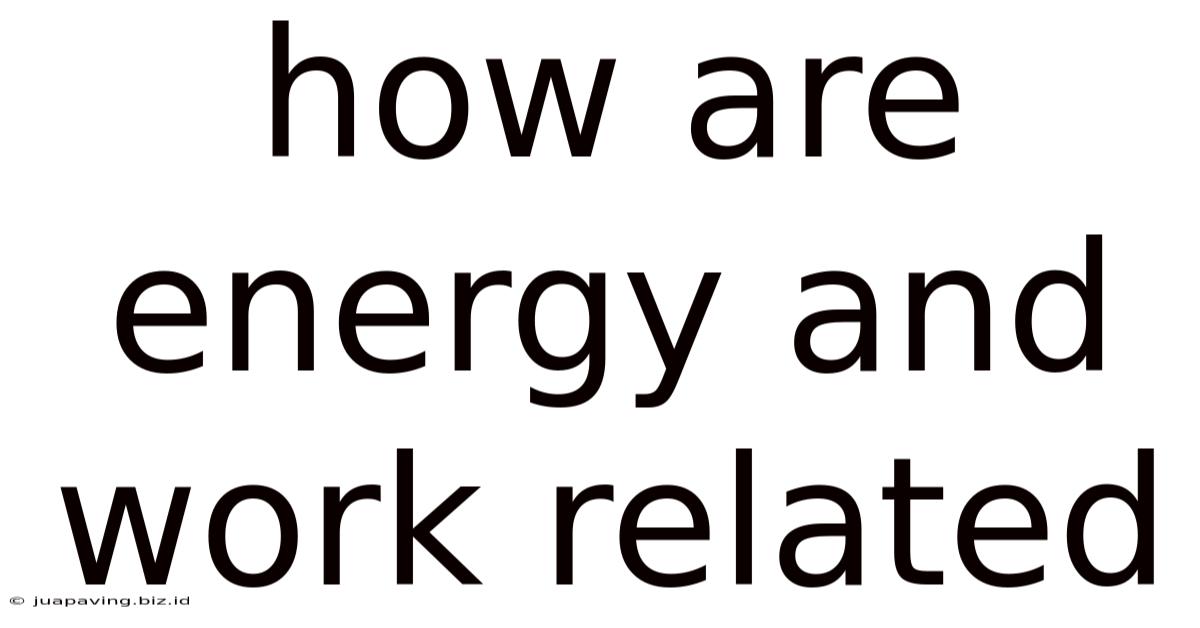
Table of Contents
How Are Energy and Work Related? A Deep Dive into Physics
Energy and work are fundamental concepts in physics, intrinsically linked in a way that governs the behavior of everything in the universe, from the smallest subatomic particles to the largest galaxies. Understanding their relationship is crucial for grasping many physical phenomena, from the simple act of lifting an object to the complexities of power generation and even the evolution of stars. This article will delve into the intricacies of this relationship, exploring the definitions, mathematical representations, different forms of energy, and real-world applications.
Defining Energy and Work
Before exploring their relationship, let's clearly define each term:
Energy: The Capacity to Do Work
Energy is a scalar quantity, meaning it only has magnitude and no direction. It's defined as the capacity of a system to do work. This means energy represents the potential of a system to cause change or exert force on its surroundings. A system with high energy has a greater potential to do work than a system with low energy. Energy is conserved; it cannot be created or destroyed, only transformed from one form to another (the First Law of Thermodynamics).
Work: The Transfer of Energy
Work, in physics, is a scalar quantity representing the transfer of energy that occurs when a force acts upon an object and causes displacement. Crucially, the force must be acting in the direction of the displacement for work to be done. If the force is perpendicular to the displacement, no work is done. For instance, carrying a heavy box horizontally involves applying a force, but no work is done because the force is perpendicular to the displacement (gravity is not considered here).
The Mathematical Relationship Between Work and Energy
The relationship between work and energy is mathematically expressed as:
Work (W) = Force (F) x Displacement (d) x cos(θ)
Where:
- W represents work, measured in Joules (J).
- F represents the force applied, measured in Newtons (N).
- d represents the displacement of the object, measured in meters (m).
- θ represents the angle between the force vector and the displacement vector.
This formula highlights the critical aspects of work: a force must be applied, and there must be movement in the direction of the force. The cosine function accounts for the angle between the force and displacement. When the force and displacement are parallel (θ = 0°), cos(θ) = 1, and the formula simplifies to W = Fd. When the force is perpendicular to the displacement (θ = 90°), cos(θ) = 0, and no work is done.
The work-energy theorem further solidifies this relationship, stating that the net work done on an object is equal to the change in its kinetic energy:
W<sub>net</sub> = ΔKE = KE<sub>final</sub> - KE<sub>initial</sub>
Where:
- W<sub>net</sub> represents the net work done.
- ΔKE represents the change in kinetic energy.
- KE<sub>final</sub> represents the final kinetic energy.
- KE<sub>initial</sub> represents the initial kinetic energy.
This theorem underscores that work is a mechanism for transferring energy. When work is done on an object, its kinetic energy changes. If positive work is done, the kinetic energy increases (the object speeds up), and if negative work is done, the kinetic energy decreases (the object slows down).
Different Forms of Energy and Their Relation to Work
Energy manifests in various forms, each capable of doing work under specific conditions:
1. Kinetic Energy: Energy of Motion
Kinetic energy is the energy an object possesses due to its motion. It's directly proportional to the object's mass and the square of its velocity:
KE = ½mv²
Where:
- KE represents kinetic energy.
- m represents the mass of the object.
- v represents the velocity of the object.
Work done on an object can increase its kinetic energy, resulting in an increase in its speed. Conversely, work can be done by an object, decreasing its kinetic energy and slowing it down. For example, a moving car possesses kinetic energy, which can do work by braking and converting that kinetic energy into heat (via friction).
2. Potential Energy: Stored Energy
Potential energy represents stored energy that can be converted into other forms of energy. Several types exist:
-
Gravitational Potential Energy: This is energy stored due to an object's position in a gravitational field. It's given by:
PE<sub>g</sub> = mgh
Where:
- PE<sub>g</sub> represents gravitational potential energy.
- m represents the mass of the object.
- g represents the acceleration due to gravity.
- h represents the height of the object above a reference point.
Lifting an object increases its gravitational potential energy. When the object falls, this potential energy is converted into kinetic energy.
-
Elastic Potential Energy: This is energy stored in an object that has been deformed, such as a stretched spring or a compressed rubber band.
-
Chemical Potential Energy: This energy is stored in the bonds between atoms and molecules. Burning fuel releases chemical potential energy, which can do work (e.g., powering a car engine).
-
Nuclear Potential Energy: This is the energy stored within the nucleus of an atom. Nuclear reactions, such as fission and fusion, release enormous amounts of energy.
In each case, the potential energy can be converted into kinetic energy or other forms of energy by doing work. For example, a stretched spring, when released, converts its elastic potential energy into kinetic energy, performing work as it moves.
3. Thermal Energy: Internal Energy of a System
Thermal energy, also known as heat, is the total kinetic energy of the particles within a system. It's related to the temperature of the system. Transferring thermal energy, often through work, can change the temperature and state of matter of a system. Friction, for example, converts kinetic energy into thermal energy (heat), demonstrating a direct link between work, kinetic energy, and thermal energy.
4. Electrical Energy: Energy of Moving Charges
Electrical energy is the energy associated with the flow of electric charge. This energy can be used to do work, for instance, powering electrical appliances. The flow of electrons through a circuit converts electrical energy into other forms, such as light, heat, or mechanical energy.
5. Radiant Energy: Energy of Electromagnetic Waves
Radiant energy is the energy carried by electromagnetic waves, such as light, radio waves, and X-rays. Solar panels convert radiant energy (sunlight) into electrical energy, which can then do work.
Real-World Applications of the Work-Energy Relationship
The relationship between work and energy is fundamental to countless real-world applications:
-
Power Generation: Power plants utilize various energy sources (chemical, nuclear, etc.) to do work, which is then converted into electricity. Hydroelectric power plants utilize the potential energy of water to generate electricity.
-
Transportation: Vehicles use the chemical potential energy of fuel to perform work, overcoming friction and accelerating the vehicle.
-
Machines: Machines are designed to perform work efficiently, converting energy from one form to another. For example, a crane utilizes electrical energy to perform work by lifting heavy objects.
-
Sports: Athletes use their stored energy (chemical potential energy) to perform work, like running or lifting weights.
-
Everyday Activities: Even simple tasks like walking or climbing stairs involve performing work, converting chemical energy into kinetic and potential energy.
Conclusion: A Unified Concept
The relationship between energy and work is a cornerstone of physics. Work represents the transfer of energy, and energy is the capacity to do work. Understanding their intricate connection is crucial for comprehending a vast range of physical phenomena and technological advancements. This fundamental principle governs the dynamics of the universe, from the smallest interactions between particles to the immense forces that shape galaxies. By grasping this relationship, we can better understand and harness the power of energy to perform work and advance our world. Further exploration into specific energy types and their applications will lead to a deeper understanding of this pivotal concept in physics.
Latest Posts
Latest Posts
-
Common Multiples Of 48 And 60
May 10, 2025
-
1500 Sq Feet To Sq Meters
May 10, 2025
-
Hexagon How Many Lines Of Symmetry
May 10, 2025
-
What Is A Bunch Of Kangaroos Called
May 10, 2025
-
Is Copper A Conductor Or Insulator
May 10, 2025
Related Post
Thank you for visiting our website which covers about How Are Energy And Work Related . We hope the information provided has been useful to you. Feel free to contact us if you have any questions or need further assistance. See you next time and don't miss to bookmark.