The Basic Unit Of Heredity Is The
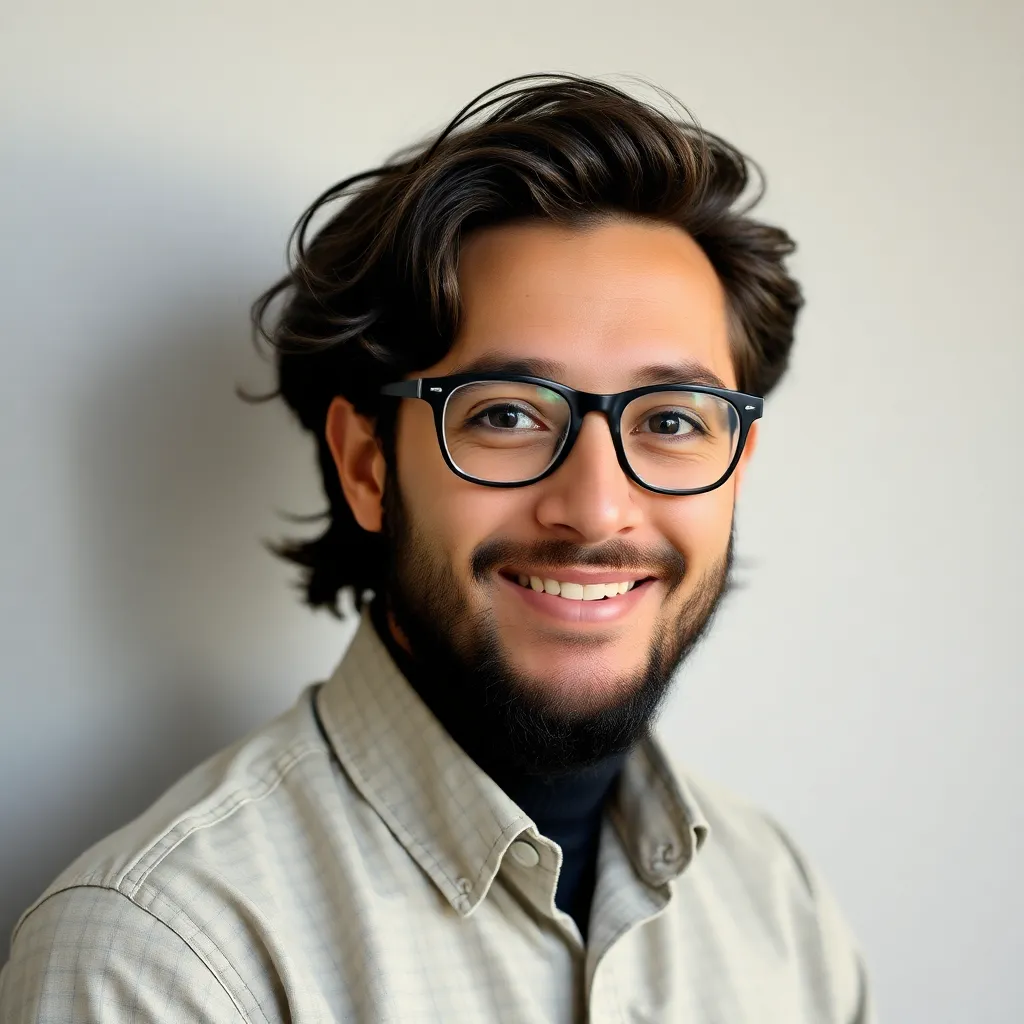
Juapaving
Apr 14, 2025 · 7 min read

Table of Contents
The Basic Unit of Heredity is the Gene: A Deep Dive into Genetics
The basic unit of heredity is the gene. This seemingly simple statement encapsulates a vast and complex field of study – genetics – that has revolutionized our understanding of life itself. From the inheritance of traits across generations to the development of groundbreaking medical treatments, the gene's role is paramount. This article delves into the intricacies of genes, exploring their structure, function, and the profound impact they have on all living organisms.
What is a Gene? A Molecular Definition
A gene is a specific sequence of deoxyribonucleic acid (DNA) that contains the instructions for building a particular protein or functional RNA molecule. Think of DNA as a vast instruction manual for life, and genes as individual chapters within that manual, each detailing the construction of a specific component. These instructions are encoded in the sequence of four nucleotide bases: adenine (A), thymine (T), guanine (G), and cytosine (C). The precise order of these bases determines the specific protein or RNA molecule that will be produced.
The Central Dogma of Molecular Biology
The process of transforming genetic information encoded in DNA into a functional protein involves a crucial two-step process known as the central dogma of molecular biology:
-
Transcription: The DNA sequence of a gene is copied into a messenger RNA (mRNA) molecule. This mRNA molecule acts as an intermediary, carrying the genetic instructions from the DNA in the nucleus to the ribosomes in the cytoplasm.
-
Translation: The mRNA molecule is "read" by ribosomes, which then use the information to assemble a chain of amino acids. This chain folds into a specific three-dimensional structure, forming a functional protein.
This protein then performs its designated role within the cell, contributing to the overall structure and function of the organism. The remarkable precision of this process ensures the faithful transmission of hereditary information from one generation to the next.
Gene Structure: Beyond the Simple Sequence
While the basic definition of a gene as a DNA sequence is accurate, the reality is far more nuanced. Genes are not simply stretches of uninterrupted coding sequences. They possess several crucial components:
-
Promoter Region: This region acts as a "switch," controlling when and how much of a gene is transcribed. Various proteins bind to the promoter, regulating gene expression based on the cell's needs and environmental cues.
-
Coding Sequence (Exons): These are the DNA sequences that actually code for the amino acid sequence of a protein. These segments are transcribed into mRNA and subsequently translated into protein.
-
Introns: These are non-coding sequences interspersed within the exons. Introns are transcribed into mRNA but are later removed before translation, a process known as splicing. The function of introns is still under investigation, but they are believed to play a role in gene regulation and evolution.
-
Terminator Sequence: This region signals the end of the gene's transcription.
The precise organization of these elements varies significantly between genes, influencing the regulation and expression of the gene's product.
Gene Function: The Orchestra of Life
Genes orchestrate virtually every aspect of an organism's life, from its physical characteristics to its susceptibility to diseases. Their functions are incredibly diverse:
-
Structural Proteins: Genes encode proteins that form the structural components of cells and tissues, such as collagen, which provides strength to connective tissues.
-
Enzymes: Many genes encode enzymes, the biological catalysts that drive countless metabolic reactions within the cell. These reactions are essential for energy production, nutrient metabolism, and countless other cellular processes.
-
Hormones: Genes direct the synthesis of hormones, chemical messengers that regulate various physiological processes, including growth, development, and reproduction.
-
Receptor Proteins: Genes produce receptor proteins, molecules that bind to signaling molecules and trigger intracellular responses. These are critical for cell communication and regulation.
-
Transcription Factors: These proteins regulate the expression of other genes, acting as master controllers of cellular processes. They often work in intricate networks, orchestrating complex developmental programs.
The intricate interplay of gene products creates a highly coordinated system ensuring the survival and reproduction of the organism.
Gene Expression: Turning Genes On and Off
Gene expression refers to the process by which the information encoded in a gene is used to synthesize a functional gene product (protein or RNA). This process is not always constant; genes can be "turned on" (expressed) or "turned off" (repressed) depending on the cell's needs and environmental conditions.
Several mechanisms regulate gene expression:
-
Transcriptional Regulation: Control of the rate of transcription, determined by the binding of transcription factors to promoter regions.
-
Post-transcriptional Regulation: Modification of mRNA molecules after transcription, affecting their stability and translation efficiency.
-
Translational Regulation: Control of the rate of protein synthesis from mRNA molecules.
-
Post-translational Regulation: Modification of proteins after translation, affecting their activity and stability.
Genetic Variation: The Engine of Evolution
Genetic variation, the differences in DNA sequences between individuals, is the raw material for evolution. These variations arise through several mechanisms:
-
Mutations: Changes in the DNA sequence, which can be caused by errors during DNA replication or exposure to mutagens. Mutations can be beneficial, harmful, or neutral, depending on their effect on gene function.
-
Gene Flow: The movement of genes between populations, which can introduce new genetic variation into a population.
-
Genetic Recombination: The shuffling of genes during sexual reproduction, which creates new combinations of alleles.
Genes and Disease: The Genetic Basis of Illness
Many diseases have a genetic component. Some diseases are caused by mutations in single genes, while others are more complex, involving multiple genes and environmental factors. Understanding the genetic basis of disease is crucial for developing effective diagnostic tools and treatments. Examples include:
-
Cystic fibrosis: Caused by mutations in the CFTR gene.
-
Sickle cell anemia: Caused by a mutation in the beta-globin gene.
-
Huntington's disease: Caused by a mutation in the HTT gene.
-
Cancer: Often caused by mutations in genes that regulate cell growth and division.
Gene Therapy: Harnessing the Power of Genes for Treatment
Gene therapy is a promising field that aims to treat diseases by modifying an individual's genes. This can involve introducing new genes into cells, correcting faulty genes, or silencing harmful genes. While still in its early stages, gene therapy holds immense potential for treating a wide range of genetic disorders and other diseases.
Epigenetics: Beyond the DNA Sequence
Epigenetics is the study of heritable changes in gene expression that do not involve alterations to the underlying DNA sequence. These changes are often caused by environmental factors, such as diet and stress, and can influence gene expression across generations. Epigenetic mechanisms include:
-
DNA methylation: The addition of methyl groups to DNA, which can repress gene expression.
-
Histone modification: Changes to the proteins around which DNA is wrapped, affecting gene accessibility.
-
Non-coding RNA: Regulatory RNA molecules that influence gene expression.
The Future of Genetics: Unraveling the Complexity of Life
Our understanding of genes is constantly evolving. Advances in genomics, the study of entire genomes, are providing unprecedented insights into the complexity of life. These advancements have led to:
-
Improved diagnostic tools: Faster and more accurate diagnosis of genetic disorders.
-
Personalized medicine: Tailored treatments based on an individual's genetic makeup.
-
Development of new therapeutic strategies: Gene therapy, CRISPR-Cas9 gene editing, and other innovative approaches.
-
Better understanding of evolution and biodiversity: Analysis of gene sequences to track evolutionary relationships and understand the mechanisms of adaptation.
In conclusion, the gene, the basic unit of heredity, is far more intricate than its simple definition suggests. Its structure, function, and regulation are the subjects of ongoing intense research, revealing the incredible complexity and beauty of life. The continued exploration of genes promises revolutionary advancements in medicine, agriculture, and our understanding of the natural world. The journey into the realm of genetics is a continuous exploration, constantly revealing new insights into the very fabric of life itself. Understanding genes is not just about understanding biology; it's about understanding ourselves.
Latest Posts
Latest Posts
-
How Many Meters In 1 5 Kilometers
May 09, 2025
-
The Vertebral Column Extends From The Skull To The
May 09, 2025
-
The Physical Expression Of An Organisms Genes
May 09, 2025
-
Which Of The Following Is Derived Unit
May 09, 2025
-
What Is The Greatest Common Factor Of 6 And 16
May 09, 2025
Related Post
Thank you for visiting our website which covers about The Basic Unit Of Heredity Is The . We hope the information provided has been useful to you. Feel free to contact us if you have any questions or need further assistance. See you next time and don't miss to bookmark.