Oxidation Reduction Reactions In Cellular Respiration
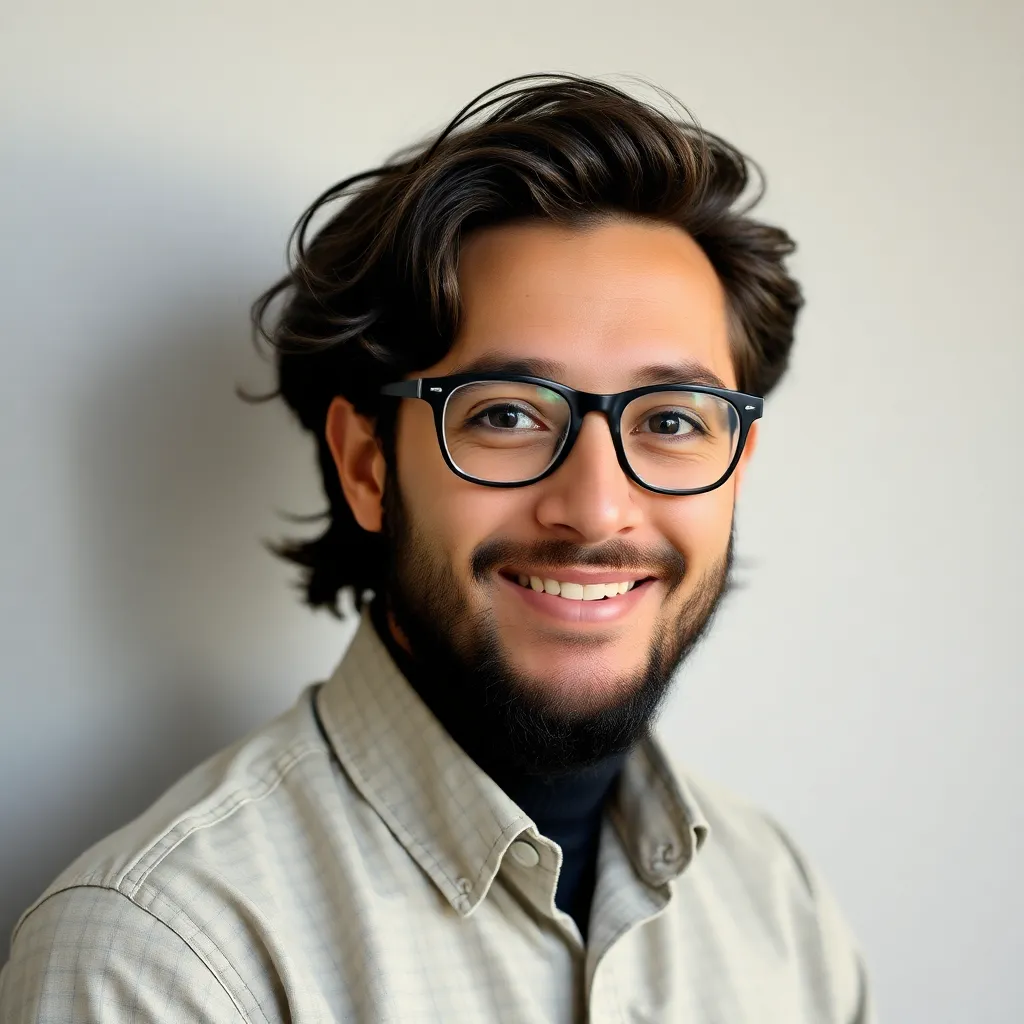
Juapaving
Mar 26, 2025 · 7 min read
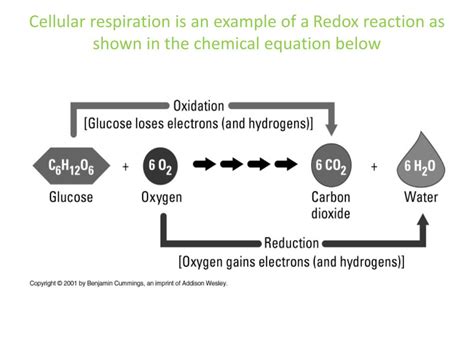
Table of Contents
Oxidation-Reduction Reactions in Cellular Respiration: The Engine of Life
Cellular respiration, the process by which cells break down glucose to generate energy in the form of ATP (adenosine triphosphate), is fundamentally driven by a series of oxidation-reduction reactions, also known as redox reactions. Understanding these redox reactions is crucial to grasping the intricate mechanisms powering life itself. This article delves deep into the role of redox reactions in the various stages of cellular respiration, exploring the electron carriers, energy transfer mechanisms, and the overall significance of this fundamental biochemical process.
What are Oxidation-Reduction Reactions?
Before we dive into the complexities of cellular respiration, let's establish a solid foundation on redox reactions. At their core, redox reactions involve the transfer of electrons between molecules.
-
Oxidation: Oxidation is the process of losing electrons. A molecule that loses electrons is said to be oxidized. Often, oxidation is accompanied by an increase in oxidation state (a measure of the degree of oxidation of an atom).
-
Reduction: Reduction is the process of gaining electrons. A molecule that gains electrons is said to be reduced. Reduction is typically associated with a decrease in oxidation state.
These two processes are always coupled; one cannot occur without the other. Whenever one molecule is oxidized, another molecule must be reduced, maintaining a balance of electron transfer. This is why they are often referred to as redox reactions.
Cellular Respiration: A Redox Symphony
Cellular respiration is a multi-step process that can be broadly divided into four main stages:
- Glycolysis: The breakdown of glucose in the cytoplasm.
- Pyruvate Oxidation: The conversion of pyruvate to acetyl-CoA in the mitochondrial matrix.
- Citric Acid Cycle (Krebs Cycle): A cyclic series of reactions in the mitochondrial matrix.
- Oxidative Phosphorylation: Electron transport and chemiosmosis in the inner mitochondrial membrane.
Each stage involves intricate redox reactions that contribute to the overall energy yield of the process.
1. Glycolysis: The Initial Steps
Glycolysis, the first stage of cellular respiration, occurs in the cytoplasm and doesn't directly require oxygen. It's a ten-step pathway that begins with glucose and ends with two molecules of pyruvate. While not as heavily reliant on redox reactions as subsequent stages, glycolysis still features crucial oxidation steps.
Glyceraldehyde-3-phosphate Dehydrogenase (GAPDH): A Key Redox Enzyme
The pivotal redox reaction in glycolysis occurs during the oxidation of glyceraldehyde-3-phosphate (G3P) to 1,3-bisphosphoglycerate (1,3-BPG). This reaction is catalyzed by the enzyme glyceraldehyde-3-phosphate dehydrogenase (GAPDH). In this step, G3P is oxidized, losing two electrons, which are then transferred to NAD+ (nicotinamide adenine dinucleotide), reducing it to NADH. NADH is a crucial electron carrier, playing a critical role in later stages of respiration. The energy released during this oxidation is used to phosphorylate G3P, forming a high-energy phosphate bond in 1,3-BPG.
2. Pyruvate Oxidation: Bridging Glycolysis and the Citric Acid Cycle
Pyruvate, the product of glycolysis, is transported from the cytoplasm into the mitochondrial matrix. Here, it undergoes a series of reactions known as pyruvate oxidation, which connects glycolysis to the citric acid cycle.
Decarboxylation and Oxidation
In pyruvate oxidation, pyruvate is oxidized and decarboxylated (loss of a carbon dioxide molecule). This process involves the removal of a carbon atom as CO2 and the transfer of two electrons to NAD+, reducing it to NADH. The remaining two-carbon fragment, an acetyl group, is then attached to coenzyme A (CoA), forming acetyl-CoA. Acetyl-CoA serves as the entry point for the citric acid cycle.
3. Citric Acid Cycle: The Central Hub of Redox Reactions
The citric acid cycle, also known as the Krebs cycle or tricarboxylic acid (TCA) cycle, is a central metabolic pathway within the mitochondrial matrix. It's a cyclic series of eight reactions that completely oxidize the acetyl group from acetyl-CoA, releasing CO2 as a byproduct. This cycle is characterized by a high concentration of redox reactions.
Multiple Redox Reactions and Electron Carriers
Each turn of the citric acid cycle involves multiple redox reactions, yielding several molecules of NADH and FADH2 (flavin adenine dinucleotide), another important electron carrier. For example, isocitrate dehydrogenase catalyzes the oxidation of isocitrate to α-ketoglutarate, reducing NAD+ to NADH. Similarly, α-ketoglutarate dehydrogenase oxidizes α-ketoglutarate to succinyl-CoA, generating another NADH molecule. Succinate dehydrogenase, a unique enzyme embedded in the inner mitochondrial membrane, catalyzes the oxidation of succinate to fumarate, reducing FAD (flavin adenine dinucleotide) to FADH2.
These electron carriers, NADH and FADH2, are crucial because they carry high-energy electrons to the next stage of cellular respiration: oxidative phosphorylation.
4. Oxidative Phosphorylation: The Powerhouse of the Cell
Oxidative phosphorylation is the final stage of cellular respiration, where the majority of ATP is generated. This process takes place in the inner mitochondrial membrane and involves two major components:
- Electron Transport Chain (ETC): A series of protein complexes embedded in the inner mitochondrial membrane that transfer electrons from NADH and FADH2.
- Chemiosmosis: The generation of ATP through the movement of protons across the inner mitochondrial membrane.
Electron Transport Chain: A Cascade of Redox Reactions
The ETC is a series of redox reactions where electrons from NADH and FADH2 are passed along a chain of protein complexes. Each protein complex undergoes a cycle of reduction and oxidation, with electrons moving from a higher energy level to a lower energy level. This electron flow releases energy, which is used to pump protons (H+) from the mitochondrial matrix to the intermembrane space, creating a proton gradient. Oxygen (O2) acts as the final electron acceptor in the ETC, becoming reduced to water (H2O). Without oxygen, the electron transport chain would halt, significantly reducing ATP production.
Chemiosmosis: Harnessing the Proton Gradient
The proton gradient established by the ETC is crucial for ATP synthesis. Protons flow back into the mitochondrial matrix through ATP synthase, a protein complex that acts as a molecular turbine. The flow of protons drives the rotation of ATP synthase, which catalyzes the synthesis of ATP from ADP (adenosine diphosphate) and inorganic phosphate (Pi). This process is known as chemiosmosis, and it's the major source of ATP production in cellular respiration.
The Significance of Redox Reactions in Cellular Respiration
Redox reactions are fundamental to the efficient energy extraction from glucose during cellular respiration. The sequential transfer of electrons from glucose to oxygen through the various electron carriers (NADH, FADH2) allows for the controlled release of energy, preventing a sudden, uncontrolled burst of energy that could damage the cell. The controlled release of energy through redox reactions enables the cell to efficiently synthesize large quantities of ATP, which powers numerous cellular processes.
Beyond Cellular Respiration: Redox Reactions in Other Biological Processes
The significance of redox reactions extends far beyond cellular respiration. Many other essential biological processes rely on redox reactions, including:
- Photosynthesis: The process by which plants convert light energy into chemical energy. Redox reactions are central to the light-dependent and light-independent reactions.
- Nitrogen fixation: The conversion of atmospheric nitrogen (N2) to ammonia (NH3), a process essential for plant growth. This involves redox reactions catalyzed by nitrogenase enzymes.
- Metabolism of lipids and proteins: The breakdown and synthesis of lipids and proteins also involve various redox reactions.
- Antioxidant defense systems: Antioxidants protect cells from damage caused by reactive oxygen species (ROS), which are highly reactive molecules generated during redox reactions.
Conclusion: The Importance of Redox Balance
Oxidation-reduction reactions are fundamental to life, playing a critical role in energy production, metabolic pathways, and maintaining cellular homeostasis. Understanding the intricacies of these reactions in cellular respiration is crucial for comprehending how living organisms derive energy from their food sources. Furthermore, appreciating the broader context of redox reactions in other biological processes illuminates the fundamental importance of redox balance in maintaining life itself. Imbalances in redox reactions can lead to oxidative stress, contributing to various diseases and aging. Therefore, a deep understanding of these processes remains essential in biomedical research and biotechnology.
Latest Posts
Latest Posts
-
What Is The Lcm For 3 And 4
Mar 29, 2025
-
What Percent Of 2 Is 3
Mar 29, 2025
-
Which Organelle Is Found Only In Plant Cells
Mar 29, 2025
-
What Is One Eighth As A Percentage
Mar 29, 2025
-
Solve X 3 1 7 15
Mar 29, 2025
Related Post
Thank you for visiting our website which covers about Oxidation Reduction Reactions In Cellular Respiration . We hope the information provided has been useful to you. Feel free to contact us if you have any questions or need further assistance. See you next time and don't miss to bookmark.