How Many Rna Polymerases Are Found In Prokaryotes
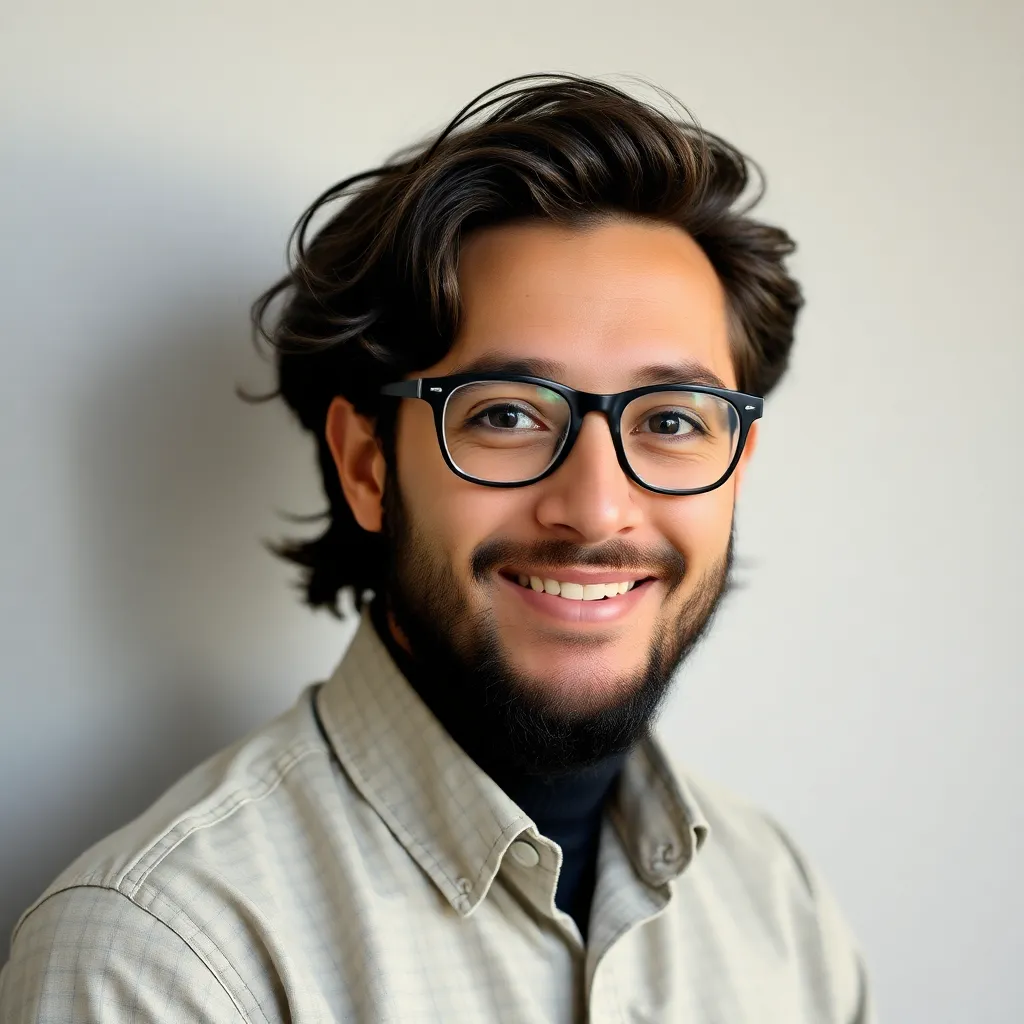
Juapaving
Apr 01, 2025 · 6 min read
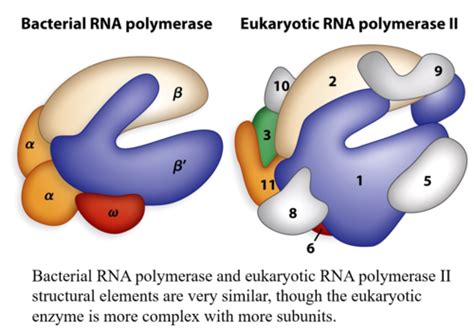
Table of Contents
How Many RNA Polymerases Are Found in Prokaryotes? A Deep Dive into Transcription
The process of transcription, where DNA is used as a template to synthesize RNA, is fundamental to life. Understanding the machinery involved is crucial to grasping the intricacies of gene expression and regulation. While eukaryotes boast a complex transcriptional apparatus with multiple RNA polymerases, prokaryotes exhibit a surprising simplicity, relying primarily on a single, versatile enzyme. This article delves deep into the world of prokaryotic transcription, exploring the single RNA polymerase, its structure, function, and the intricacies of how it orchestrates gene expression in these organisms.
The Simplicity and Efficiency of a Single RNA Polymerase
Unlike eukaryotes, which employ distinct RNA polymerases for synthesizing different RNA types (mRNA, rRNA, tRNA), prokaryotes utilize a single core RNA polymerase to transcribe all their genes. This seemingly simple arrangement belies a sophisticated enzyme capable of performing a wide array of tasks with remarkable efficiency. This streamlined approach reflects the overall efficiency observed in prokaryotic cellular processes, where rapid growth and adaptation are prioritized.
This singular enzyme, often referred to as bacterial RNA polymerase, is a holoenzyme—a multi-subunit complex—composed of several essential components crucial for its function. Understanding its composition is key to understanding its remarkable capabilities.
The Core Enzyme and its Subunits
The core enzyme of prokaryotic RNA polymerase comprises five subunits:
-
Two α (alpha) subunits: These subunits play crucial roles in enzyme assembly and interactions with regulatory proteins. They're essential for promoter recognition and the formation of the functional holoenzyme.
-
One β (beta) subunit: This subunit is the primary catalytic subunit, responsible for the actual polymerization of ribonucleotides during RNA synthesis. Its active site binds to the incoming ribonucleoside triphosphates (NTPs) and catalyzes the formation of phosphodiester bonds.
-
One β' (beta-prime) subunit: This subunit, along with the β subunit, forms the catalytic core. It also contributes to DNA binding and maintaining the stability of the enzyme-DNA complex.
-
One ω (omega) subunit: This subunit plays a role in enzyme assembly and stability, influencing the overall structural integrity and possibly the efficiency of transcription initiation. Its exact function remains an active area of research.
This core enzyme (α₂ββ'ω) is capable of RNA synthesis, but it lacks the specificity to initiate transcription at the correct sites on the DNA. That's where the sigma factor comes in.
The Sigma Factor: Specificity and Promoter Recognition
The sigma factor (σ) is a crucial regulatory subunit that binds to the core enzyme, forming the RNA polymerase holoenzyme (α₂ββ'ωσ). The sigma factor is not directly involved in the catalytic process of polymerization but is absolutely essential for initiating transcription. Its primary role is to confer promoter specificity to the enzyme.
Promoters are specific DNA sequences located upstream of genes that signal the starting point of transcription. Different sigma factors recognize different promoter sequences, thereby regulating the expression of different sets of genes. This allows for a highly controlled and flexible system of gene expression based on environmental stimuli or developmental needs.
Different bacterial species might possess a variety of sigma factors, each with slightly different recognition sequences, ensuring a highly nuanced regulation of gene expression. This is particularly significant in bacteria responding to environmental stress, infection, or development.
Beyond the Core: Accessory Factors and Transcription Regulation
While the core enzyme and sigma factor are the fundamental components of prokaryotic RNA polymerase, various accessory proteins can modulate its activity, influencing the overall rate and fidelity of transcription. These factors contribute to the intricate regulation of gene expression in response to various cellular signals.
Some accessory factors can enhance or impede transcription initiation, while others influence the elongation or termination phases of transcription. Their involvement highlights the sophisticated control mechanisms underlying prokaryotic gene expression, reflecting the adaptability of these organisms to changing environments.
Transcription Initiation: A Multi-Step Process
Initiation of transcription is a complex, tightly regulated process. The holoenzyme first binds to the promoter region of the DNA, forming a closed complex. The enzyme then unwinds the DNA at the promoter, forming an open complex, exposing the template strand. The first ribonucleotide is then incorporated, initiating RNA synthesis. After a few nucleotides have been added, the sigma factor typically dissociates from the core enzyme, which then continues with the elongation phase.
Transcription Elongation: Synthesizing the RNA Chain
Once the initiation phase is complete, the core enzyme proceeds along the DNA template, synthesizing the RNA molecule. This process is called elongation. The enzyme unwinds the DNA ahead of it and rewinds it behind it, maintaining a transcription bubble. The newly synthesized RNA molecule is progressively released from the enzyme as elongation proceeds.
Elongation is a highly processive event. Various elongation factors can enhance the processivity and stability of the transcription complex, ensuring efficient and accurate RNA synthesis. These factors play a critical role in mediating the interactions between the polymerase and the DNA template, ensuring that the enzyme does not dissociate prematurely.
Transcription Termination: Ending the Process
Transcription termination marks the end of RNA synthesis. There are two main mechanisms of transcription termination in prokaryotes:
-
Rho-independent termination: This mechanism relies on specific sequences within the RNA molecule that form hairpin structures. These structures cause the RNA polymerase to pause and dissociate from the DNA template.
-
Rho-dependent termination: This mechanism requires the participation of the Rho protein, a hexameric ATPase. Rho protein binds to the RNA transcript and moves towards the RNA polymerase, causing it to dissociate from the DNA.
Variations and Exceptions: Beyond the Typical Single Polymerase
While the vast majority of prokaryotes utilize a single RNA polymerase for all their transcriptional needs, some exceptions and variations exist. Certain bacteria might possess specialized RNA polymerases with unique functions or modified subunits that contribute to the diversity of transcriptional regulation in these organisms. These specializations often reflect unique metabolic or environmental adaptations. Further research is needed to fully understand the range of functions and regulation in these diverse bacterial systems.
Conclusion: The Power of Simplicity in Prokaryotic Transcription
The use of a single RNA polymerase in prokaryotes exemplifies the remarkable efficiency and elegance of their transcriptional machinery. This single enzyme, through its interaction with various sigma factors and accessory proteins, orchestrates the precise and controlled expression of thousands of genes. This system provides a highly dynamic and adaptive mechanism for responding to environmental changes and coordinating cellular processes. While seemingly simple, the prokaryotic transcriptional system exhibits a level of complexity and fine-tuning that underscores the fundamental importance of this crucial process in life. Ongoing research continues to reveal further intricacies of this system, highlighting the continuing importance of understanding prokaryotic transcription for various applications in biotechnology and medicine. The remarkable adaptability of this system also holds valuable lessons for synthetic biology, engineering novel transcriptional systems with customized characteristics.
Latest Posts
Latest Posts
-
Which Base Is Found Only In Rna
Apr 02, 2025
-
Difference Between Meiosis 1 And Meiosis 2
Apr 02, 2025
-
Equation Of Circle In Parametric Form
Apr 02, 2025
-
How To Find The Complement Of An Angle
Apr 02, 2025
-
Nice Word That Starts With E
Apr 02, 2025
Related Post
Thank you for visiting our website which covers about How Many Rna Polymerases Are Found In Prokaryotes . We hope the information provided has been useful to you. Feel free to contact us if you have any questions or need further assistance. See you next time and don't miss to bookmark.