An Example Of A Longitudinal Wave
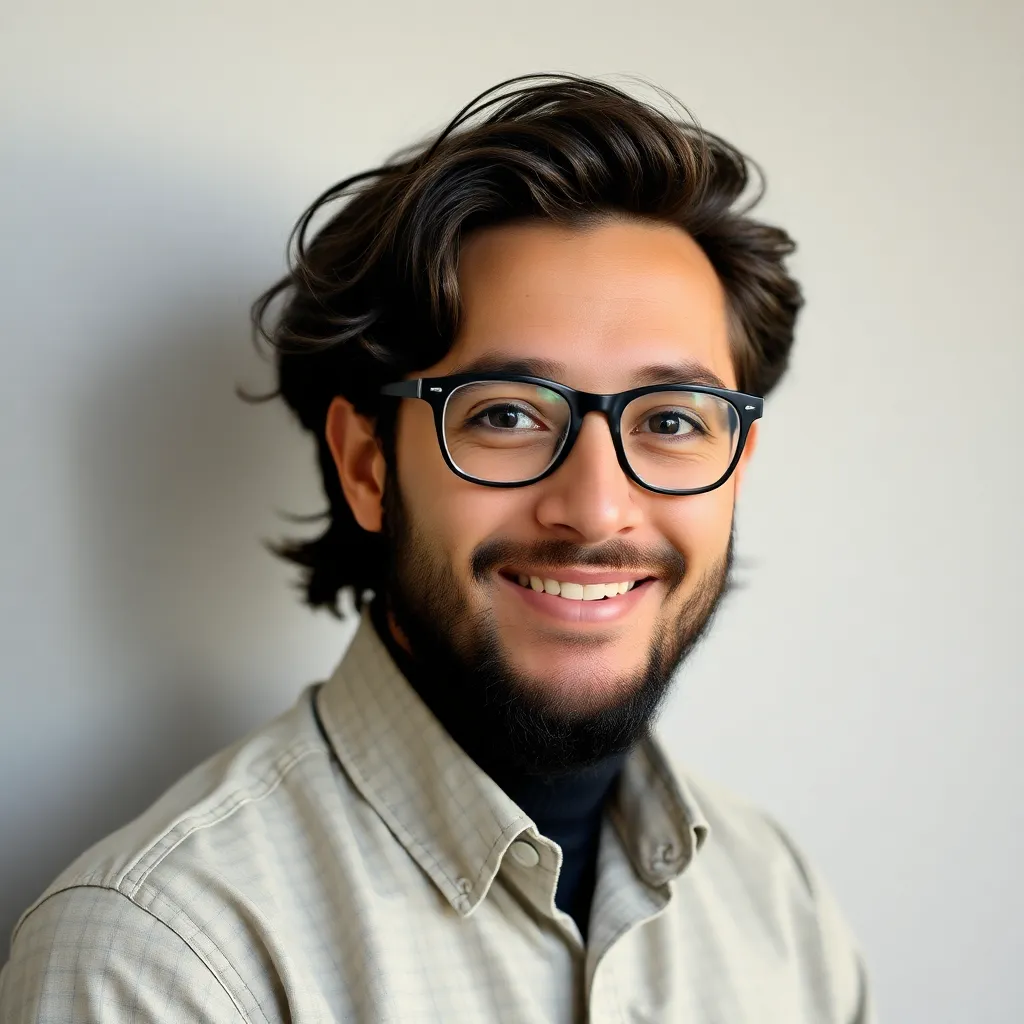
Juapaving
May 09, 2025 · 6 min read
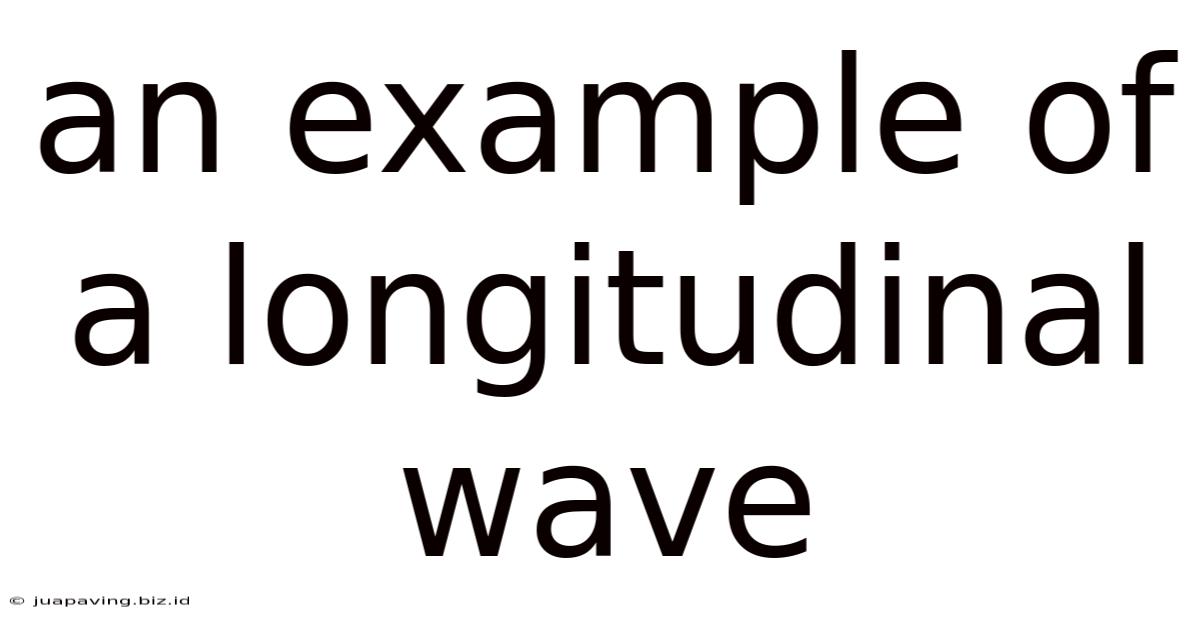
Table of Contents
An Example of a Longitudinal Wave: Sound Waves and Their Properties
Longitudinal waves are a fascinating area of physics, representing a fundamental type of wave motion. Understanding these waves is key to grasping numerous phenomena, from the way we hear to the functioning of medical imaging technologies. This article will delve deep into the characteristics of longitudinal waves, using the ubiquitous example of sound waves to illustrate their properties and behavior. We'll explore their generation, propagation, and interaction with matter, providing a comprehensive understanding of this vital wave type.
What are Longitudinal Waves?
Before we dive into the specifics of sound, let's define what constitutes a longitudinal wave. Unlike transverse waves, where the oscillation is perpendicular to the direction of energy transfer (think of a wave on a string), longitudinal waves involve oscillations that occur parallel to the direction of energy propagation. Imagine pushing a spring: the compression and rarefaction travel along the spring's length – that's a longitudinal wave in action.
Key characteristics of longitudinal waves include:
- Compression and Rarefaction: These are the defining features. Compressions are regions of high particle density, where the medium is squeezed together. Rarefactions are regions of low particle density, where the medium is spread out. These alternating regions of compression and rarefaction propagate through the medium.
- Wavelength (λ): The distance between two successive compressions (or two successive rarefactions) is defined as the wavelength. It's a measure of the wave's spatial extent.
- Frequency (f): This represents the number of complete wave cycles (one compression and one rarefaction) passing a given point per unit time, typically measured in Hertz (Hz). Higher frequency means more cycles per second, and consequently, higher energy.
- Amplitude: This refers to the maximum displacement of particles from their equilibrium position. A higher amplitude signifies a louder sound (in the case of sound waves) or a stronger wave in general.
- Speed (v): The speed of a longitudinal wave depends on the properties of the medium through which it travels. For example, the speed of sound differs in air, water, and solids.
Sound Waves: The Perfect Example of Longitudinal Waves
Sound waves are a prime example of longitudinal waves. They are created by the vibration of an object, which causes the surrounding air molecules to oscillate back and forth along the same direction as the wave's propagation. These oscillations create alternating regions of compression and rarefaction that travel outwards from the source.
How Sound Waves are Generated
Consider striking a tuning fork. The prongs vibrate, pushing the adjacent air molecules together (compression). As the prongs move back, the molecules spread out (rarefaction). This cyclical compression and rarefaction creates a longitudinal wave that propagates through the air. The frequency of the wave corresponds to the frequency of the tuning fork's vibration, determining the pitch of the sound. The amplitude of the vibration corresponds to the intensity or loudness of the sound.
Propagation of Sound Waves through Different Media
The speed of sound differs dramatically depending on the medium. This is because the speed depends on the properties of the medium, primarily its density and elasticity. Sound travels fastest in solids, slower in liquids, and slowest in gases. This is because the molecules in solids are closer together and interact more strongly than in liquids or gases, allowing the compression and rarefaction to travel more efficiently.
- Solids: The strong intermolecular forces in solids allow for rapid transmission of the compression and rarefaction, resulting in high sound speeds.
- Liquids: Liquids have weaker intermolecular forces than solids, resulting in slower sound speeds.
- Gases: Gases have weak intermolecular forces and low density, leading to the slowest sound speeds. The speed of sound in air is significantly affected by factors like temperature and humidity.
The Role of Density and Elasticity
Density plays a crucial role because denser mediums offer greater resistance to the propagation of the wave. Higher density means that the molecules are packed more tightly together, making it harder for the wave to propagate.
Elasticity, on the other hand, refers to the ability of the medium to return to its original shape after being deformed. A more elastic medium will allow for faster propagation of the compression and rarefaction, as it can more easily recover from the disturbance. The interplay between density and elasticity ultimately determines the speed of sound in a given medium.
Interaction of Sound Waves with Matter
Sound waves interact with matter in several ways, leading to various phenomena:
- Reflection: When a sound wave encounters a surface, it can bounce back, a phenomenon known as reflection. This is how echoes are formed. The angle of incidence (the angle at which the wave hits the surface) equals the angle of reflection.
- Refraction: Sound waves can bend when they pass from one medium to another, a process called refraction. This occurs because the speed of sound differs in different media. The bending of sound waves is influenced by the change in the speed of sound across the media boundary.
- Diffraction: Sound waves can bend around obstacles. This phenomenon, known as diffraction, is more pronounced when the wavelength is comparable to the size of the obstacle. A larger wavelength leads to greater diffraction.
- Interference: When two or more sound waves overlap, they interfere with each other. Constructive interference occurs when the waves are in phase, resulting in a louder sound. Destructive interference occurs when the waves are out of phase, leading to a quieter or even silent sound. This is the principle behind noise-canceling technology.
- Absorption: Sound waves can lose energy as they travel through a medium. This is known as absorption. The extent of absorption depends on the material's properties and the frequency of the sound wave. Soft materials tend to absorb more sound than hard materials.
Applications of Understanding Longitudinal Waves
The understanding of longitudinal waves, particularly sound waves, has numerous practical applications:
- Medical Ultrasound: Ultrasound imaging uses high-frequency sound waves to create images of internal organs and tissues. The reflection and scattering of sound waves provide information about the structures within the body.
- Sonar: Sonar (Sound Navigation and Ranging) utilizes sound waves to detect objects underwater. It's widely used in navigation, fishing, and oceanographic research.
- Seismic Waves: Seismic waves, which are longitudinal (P-waves) and transverse (S-waves), are used to study the Earth's interior. The propagation of these waves through the Earth reveals information about the planet's structure and composition.
- Music and Acoustics: The understanding of sound waves is fundamental to the creation and appreciation of music. Acoustics involves the study of sound waves and their behavior in enclosed spaces, impacting the design of concert halls and recording studios.
- Architectural Acoustics: The principles of sound wave reflection, absorption, and interference are critical in architectural design to optimize sound quality in spaces like concert halls and theaters. Proper design minimizes unwanted echoes and reverberations.
Conclusion
Longitudinal waves, particularly sound waves, are a fundamental aspect of physics with far-reaching implications. This article has explored the characteristics, generation, propagation, and interactions of longitudinal waves, using the readily understandable example of sound. From understanding the basics of pitch and loudness to the sophisticated applications in medical imaging and geological surveys, mastering the concepts of longitudinal waves is crucial for understanding our world. Further exploration into specific areas like the Doppler effect and the mathematical representation of sound waves will provide even deeper insights into the fascinating world of longitudinal wave phenomena. The importance of these waves in everyday life and advanced technologies is undeniable, highlighting the significance of continuous research and development in this field.
Latest Posts
Latest Posts
-
What Is The Difference Between A Prism And Pyramid
May 11, 2025
-
Subject Verb Agreement Worksheet Class 7
May 11, 2025
-
Which Is A Limitation Of Scientific Management
May 11, 2025
-
Words That Start And End With V
May 11, 2025
-
What Is Lcm Of 7 And 12
May 11, 2025
Related Post
Thank you for visiting our website which covers about An Example Of A Longitudinal Wave . We hope the information provided has been useful to you. Feel free to contact us if you have any questions or need further assistance. See you next time and don't miss to bookmark.