Why Is The Shape Of Proteins Important
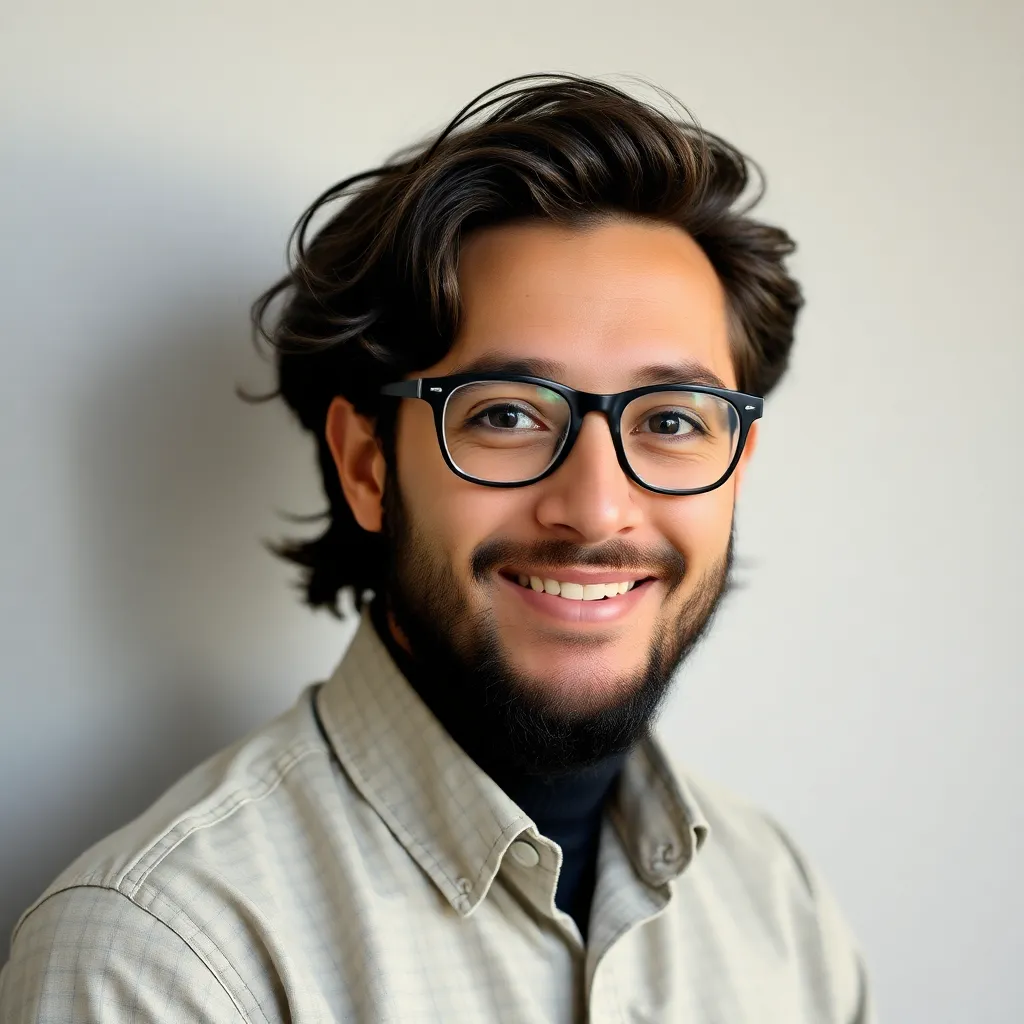
Juapaving
May 12, 2025 · 7 min read

Table of Contents
Why is the Shape of Proteins Important? A Deep Dive into Protein Structure and Function
Proteins are the workhorses of life. These complex molecules perform a vast array of functions, from catalyzing biochemical reactions to providing structural support and facilitating cell signaling. But how do they manage such diverse roles? The answer lies in their shape. The three-dimensional structure of a protein, its conformation, is inextricably linked to its function. A slight alteration in shape can drastically affect, or even completely abolish, its ability to perform its designated task. This article delves deep into the crucial importance of protein shape, exploring the different levels of protein structure and how they contribute to overall function.
The Four Levels of Protein Structure: A Hierarchical Organization
Understanding the importance of protein shape necessitates understanding the different levels of protein structure. These levels build upon each other, culminating in the unique three-dimensional conformation that dictates a protein's function.
1. Primary Structure: The Amino Acid Sequence
The primary structure of a protein is simply its amino acid sequence. This linear chain of amino acids, linked together by peptide bonds, is dictated by the genetic code encoded within DNA. Each amino acid possesses unique properties – some are hydrophobic (water-repelling), others are hydrophilic (water-attracting), and some carry a positive or negative charge. This variation in properties is critical because it determines how the polypeptide chain will fold into its higher-order structures. Even a single amino acid substitution can have profound consequences, as famously demonstrated in sickle cell anemia where a single amino acid change in hemoglobin alters its shape and function.
2. Secondary Structure: Local Folding Patterns
The primary structure begins to fold into local, repeating patterns, forming the secondary structure. These patterns are stabilized by hydrogen bonds between the backbone atoms of the amino acid chain. The most common secondary structures are:
-
α-helices: A right-handed coiled structure stabilized by hydrogen bonds between the carbonyl oxygen of one amino acid and the amide hydrogen of an amino acid four residues down the chain. The R-groups (side chains) of the amino acids project outwards from the helix.
-
β-sheets: Formed by extended segments of the polypeptide chain arranged side-by-side, forming a pleated sheet-like structure. Hydrogen bonds form between the carbonyl oxygen and amide hydrogen of adjacent strands. β-sheets can be parallel (strands running in the same direction) or antiparallel (strands running in opposite directions).
The arrangement and proportion of α-helices and β-sheets within a protein contribute significantly to its overall shape and properties.
3. Tertiary Structure: The 3D Arrangement of a Polypeptide Chain
The tertiary structure refers to the overall three-dimensional arrangement of a single polypeptide chain. This structure is determined by various interactions between the amino acid side chains (R-groups), including:
-
Hydrophobic interactions: Nonpolar side chains cluster together in the protein's interior, minimizing their contact with water.
-
Hydrogen bonds: Form between polar side chains and other polar groups within the protein.
-
Ionic bonds (salt bridges): Form between oppositely charged side chains.
-
Disulfide bonds: Covalent bonds formed between cysteine residues, strongly stabilizing the protein's structure.
The tertiary structure is crucial because it brings specific amino acid residues into close proximity, creating active sites for enzymes, binding sites for ligands, and other functional regions. The precise folding is crucial for functionality; misfolding can lead to inactive or malfunctioning proteins.
4. Quaternary Structure: Multiple Polypeptide Chains
Some proteins consist of multiple polypeptide chains, also known as subunits, which associate to form the quaternary structure. These subunits can be identical or different. Interactions between the subunits, similar to those in tertiary structure, maintain the quaternary structure. Hemoglobin, for example, comprises four subunits that work together to bind and transport oxygen. The quaternary structure allows for cooperative binding and allosteric regulation, enhancing the protein's functionality.
The Impact of Shape on Protein Function: A Multifaceted Relationship
The precise three-dimensional structure of a protein is paramount to its function. Even small changes in shape can dramatically alter or abolish its activity. This relationship manifests in several ways:
Enzyme Activity: Substrate Binding and Catalysis
Enzymes are biological catalysts that accelerate biochemical reactions. Their active sites, specifically shaped regions on the enzyme's surface, are designed to bind specific substrates (reactants). The shape of the active site is complementary to the shape of the substrate, like a lock and key. This precise binding is essential for catalysis to occur. Any alteration in the enzyme's shape could disrupt substrate binding and inhibit catalytic activity.
Receptor Binding: Cell Signaling and Communication
Receptors are proteins that bind to specific molecules (ligands), triggering cellular responses. The shape of the receptor's ligand-binding site is highly specific to its ligand. A change in receptor shape could prevent ligand binding, hindering cellular signaling pathways and impacting cell function. This is crucial in processes like hormone action, neurotransmission, and immune responses.
Structural Proteins: Providing Support and Stability
Structural proteins provide mechanical support and maintain cellular shape. Collagen, for example, is a fibrous protein with a triple helix structure providing strength and flexibility to connective tissues. The specific shape of collagen fibers is vital for maintaining tissue integrity. Any change in its shape could compromise the structural integrity of tissues.
Transport Proteins: Facilitating Molecular Movement
Transport proteins facilitate the movement of molecules across cell membranes. These proteins have specific binding sites for their target molecules. The shape of the binding site and the conformational changes undergone by the protein during transport are essential for efficient and regulated movement of molecules. Changes in protein shape could disrupt transport, leading to metabolic imbalances.
Motor Proteins: Driving Cellular Movement
Motor proteins like myosin and kinesin are responsible for cellular movement. Their specific shapes and ability to undergo conformational changes, driven by ATP hydrolysis, allow them to move along cytoskeletal filaments, facilitating cellular processes like muscle contraction and intracellular transport. Changes in shape are integral to their “stepping” mechanism, and any disruptions would halt these vital functions.
Factors Affecting Protein Shape and Stability
Several factors influence a protein's shape and stability:
-
Temperature: High temperatures can disrupt weak interactions (hydrogen bonds, hydrophobic interactions) that maintain protein structure, leading to denaturation (loss of shape and function).
-
pH: Changes in pH can alter the charge of amino acid side chains, affecting ionic interactions and protein stability.
-
Chemicals: Certain chemicals, like detergents or chaotropic agents, can disrupt protein structure.
-
Mutations: Changes in the amino acid sequence (genetic mutations) can alter the protein's shape and function, potentially leading to disease.
-
Post-translational modifications: Chemical modifications to amino acids after protein synthesis can impact protein shape and function.
Understanding these factors is crucial in fields like drug discovery, where researchers aim to design molecules that specifically interact with proteins to modulate their activity.
The Significance of Protein Misfolding and Aggregation
When proteins fail to fold correctly, they can lead to serious consequences. Misfolded proteins can be inactive, or worse, they can aggregate, forming clumps that interfere with cellular function. This is implicated in many diseases, including Alzheimer's disease, Parkinson's disease, and cystic fibrosis. The aggregation of misfolded proteins can disrupt cellular processes and lead to cell death.
Conclusion: Shape Defines Function
In conclusion, the shape of a protein is intrinsically linked to its function. The precise arrangement of amino acids, from the primary sequence to the quaternary structure, dictates a protein's ability to perform its specific role. Understanding this intricate relationship is vital for comprehending fundamental biological processes and tackling diseases related to protein misfolding and dysfunction. From enzyme activity and receptor binding to structural support and molecular transport, the shape of a protein is the key to its life-sustaining function. Continued research into protein structure and function promises breakthroughs in treating a wide range of diseases and developing new technologies based on protein engineering.
Latest Posts
Related Post
Thank you for visiting our website which covers about Why Is The Shape Of Proteins Important . We hope the information provided has been useful to you. Feel free to contact us if you have any questions or need further assistance. See you next time and don't miss to bookmark.