Why Does Carbon Form Covalent Bonds
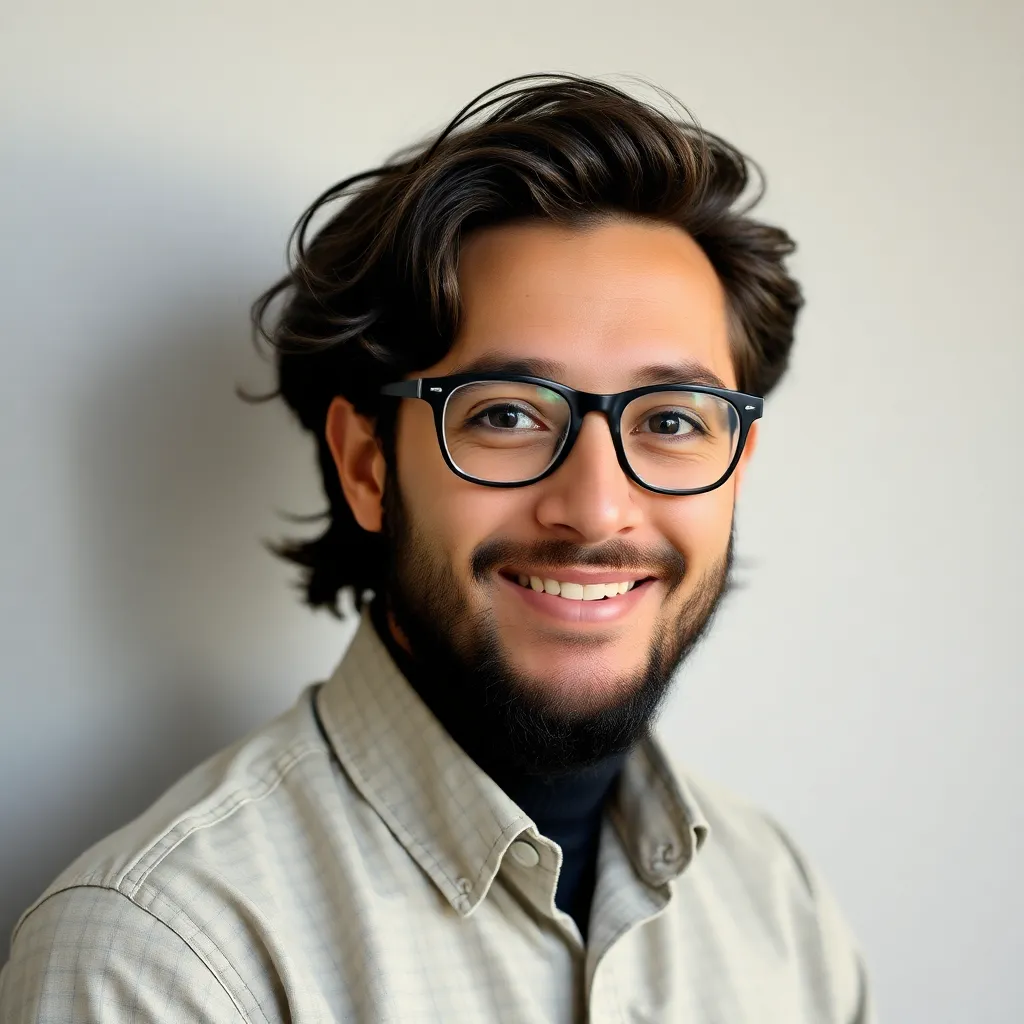
Juapaving
Apr 13, 2025 · 6 min read

Table of Contents
Why Does Carbon Form Covalent Bonds? The Extraordinary Chemistry of Carbon
Carbon, the backbone of life and the foundation of countless materials, boasts a unique and fascinating chemistry. Its ability to form incredibly diverse and complex molecules is largely due to its predilection for forming covalent bonds. But why? This article delves deep into the electronic structure of carbon and explores the reasons behind its strong preference for covalent bonding, examining the implications for organic chemistry and materials science.
The Electronic Structure: The Key to Understanding Carbon's Bonding
The answer lies within carbon's electronic structure. Carbon sits in Group 14 of the periodic table, possessing six electrons in total. Its electronic configuration is 1s²2s²2p². This means it has four electrons in its outermost shell (valence shell), two in the 2s orbital and two in the 2p orbitals. This seemingly simple configuration is the key to its remarkable versatility in bonding.
The Significance of Four Valence Electrons
Having four valence electrons signifies that carbon can either gain four electrons (highly unlikely due to the significant energy required) or share four electrons with other atoms to achieve a stable octet. Gaining four electrons would create a highly unstable -4 charge, while losing four electrons to form a +4 ion is similarly energetically unfavorable.
Therefore, sharing electrons through covalent bonding emerges as the most energetically favorable and stable way for carbon to satisfy the octet rule—a fundamental principle in chemistry that states atoms tend to gain, lose, or share electrons to achieve a full outer electron shell of eight electrons.
Hybridization: Unlocking Carbon's Bonding Potential
To fully grasp carbon's bonding behaviour, we must introduce the concept of hybridization. Hybridization is the mixing of atomic orbitals within an atom to form new hybrid orbitals with different shapes and energies. In the case of carbon, the 2s and 2p orbitals hybridize to form four equivalent sp³ hybrid orbitals.
This hybridization is crucial because it allows carbon to form four strong covalent bonds with other atoms, creating a stable tetrahedral geometry. This tetrahedral arrangement is the foundation for the complex three-dimensional structures seen in organic molecules.
Types of Covalent Bonds Formed by Carbon
Carbon's ability to form covalent bonds isn't limited to the single bonds facilitated by sp³ hybridization. It can also form:
-
Double Bonds (sp² Hybridization): By hybridizing one 2s orbital with two 2p orbitals, carbon forms three sp² hybrid orbitals and leaves one unhybridized 2p orbital. This allows carbon to form three sigma bonds (single bonds) and one pi bond (a double bond involving sideways overlap of the p-orbitals), resulting in a trigonal planar geometry. Examples include the carbon-oxygen double bonds in carbonyl compounds like ketones and aldehydes, and the carbon-carbon double bonds in alkenes.
-
Triple Bonds (sp Hybridization): Carbon can hybridize one 2s orbital with only one 2p orbital, resulting in two sp hybrid orbitals and two unhybridized 2p orbitals. This configuration enables carbon to form two sigma bonds and two pi bonds (a triple bond), leading to a linear geometry. This type of bonding is characteristic of alkynes, compounds containing carbon-carbon triple bonds.
This variety in hybridization and bonding contributes significantly to the diversity of organic molecules, leading to a vast array of structures with varying properties.
The Strength of Carbon-Carbon Bonds
Another crucial factor contributing to carbon's prevalence in forming covalent bonds is the strength of carbon-carbon bonds. The bonds are remarkably strong due to the similar electronegativities of carbon atoms. Electronegativity measures an atom's tendency to attract electrons in a chemical bond. Since carbon's electronegativity is relatively similar to many other atoms, it forms strong, stable bonds without a significant ionic character. This strength allows for the creation of long chains, branched structures, and complex ring systems, all of which are essential features of organic molecules.
Implications in Organic Chemistry and Materials Science
The capacity of carbon to form strong covalent bonds with a variety of atoms—including hydrogen, oxygen, nitrogen, sulfur, and other carbon atoms—underpins the immense diversity of organic compounds. These molecules form the basis of life, comprising proteins, carbohydrates, lipids, and nucleic acids.
The Vastness of Organic Chemistry
The field of organic chemistry, which focuses on carbon-containing compounds, is vast because of the myriad ways carbon atoms can bond together. Carbon chains can be linear, branched, or cyclic. Functional groups—atoms or groups of atoms that impart specific chemical properties—can be attached to these carbon frameworks, leading to an almost limitless number of possible organic molecules.
Applications in Materials Science
The unique bonding properties of carbon also extend into materials science. The strength and stability of covalent bonds lead to the creation of materials with exceptional properties. Examples include:
-
Diamond: A network covalent solid where each carbon atom is bonded to four other carbon atoms in a tetrahedral arrangement. This results in an extremely hard, strong, and high-temperature material.
-
Graphite: A layered structure with strong covalent bonds within each layer but weaker interlayer forces. This leads to its use as a lubricant and in pencils due to the ability of layers to slide past each other.
-
Fullerenes (e.g., Buckminsterfullerene): Spherical or ellipsoidal molecules composed entirely of carbon atoms arranged in a cage-like structure. Their unique structures and properties have found applications in nanotechnology and materials science.
-
Graphene: A single layer of graphite, featuring a hexagonal lattice of carbon atoms with strong covalent bonds. It's known for its exceptional strength, conductivity, and potential applications in electronics and composites.
Comparison with Other Bonding Types
To fully appreciate carbon's preference for covalent bonds, let's briefly compare it with ionic and metallic bonding:
-
Ionic Bonds: These bonds form through the electrostatic attraction between oppositely charged ions (cations and anions). Carbon's electronic configuration makes the formation of stable ions energetically unfavorable, hence ionic bonding is not a preferred method for carbon.
-
Metallic Bonds: These bonds result from the delocalized electrons in a "sea" of electrons surrounding positively charged metal ions. Carbon does not readily form this type of bond; it lacks the necessary electronic structure for delocalized electrons.
Therefore, covalent bonding stands out as the optimal way for carbon to achieve a stable electronic configuration and to form a wide variety of strong and stable compounds.
Conclusion: The Remarkable Versatility of Carbon
The remarkable versatility of carbon in forming covalent bonds is a testament to its unique electronic structure and the strength of the resulting bonds. This property is fundamental to organic chemistry, materials science, and even the existence of life itself. Understanding the reasons behind carbon's preference for covalent bonding provides crucial insight into the vast world of organic molecules and the remarkable diversity of materials based on this fascinating element. From the intricate molecules of life to the cutting-edge materials of modern technology, the story of carbon's covalent bonding continues to unfold, promising new discoveries and innovations for years to come. The continuing research into carbon-based materials highlights the ongoing importance of understanding this fundamental aspect of its chemistry. The future of materials science and nanotechnology heavily relies on the continued exploration of carbon's impressive bonding capabilities.
Latest Posts
Latest Posts
-
How To Find Percent By Weight
Apr 15, 2025
-
How Many Feet In 160 Inches
Apr 15, 2025
-
What Is The Most Basic Unit Of Life
Apr 15, 2025
-
What Is The Answer To Multiplication Called
Apr 15, 2025
-
The Male Part Of The Flower Is Called
Apr 15, 2025
Related Post
Thank you for visiting our website which covers about Why Does Carbon Form Covalent Bonds . We hope the information provided has been useful to you. Feel free to contact us if you have any questions or need further assistance. See you next time and don't miss to bookmark.