Why Do Insulators Not Conduct Electricity
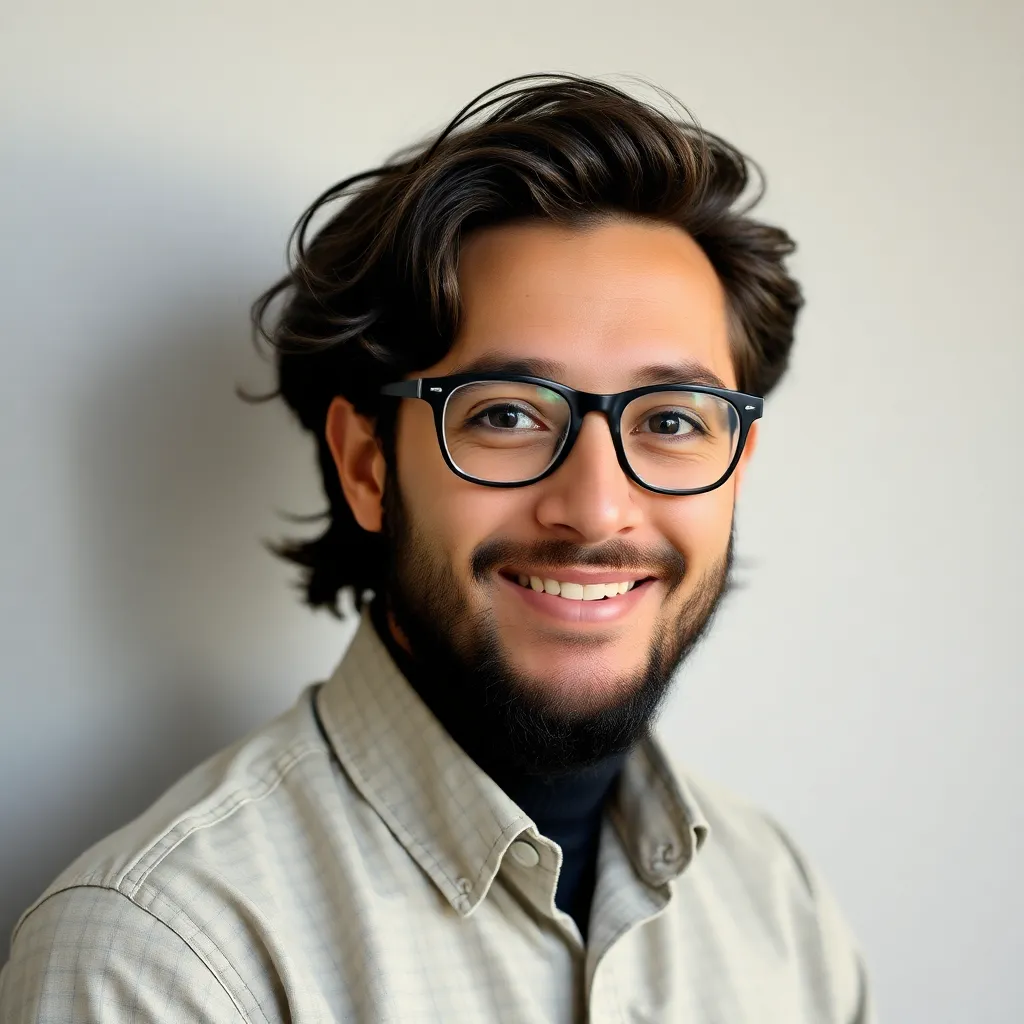
Juapaving
Apr 24, 2025 · 6 min read

Table of Contents
Why Do Insulators Not Conduct Electricity? A Deep Dive into Atomic Structure and Electron Behavior
Understanding why insulators don't conduct electricity requires delving into the fascinating world of atomic structure and electron behavior. While conductors readily allow the flow of electric current, insulators strongly resist it. This fundamental difference boils down to the availability and mobility of electrons within the material's atomic structure. This article will explore this difference in detail, examining the role of valence electrons, band gaps, and other key factors that determine a material's electrical conductivity.
The Role of Valence Electrons: The Key Players in Conductivity
Atoms are composed of a nucleus containing protons and neutrons, surrounded by orbiting electrons. The electrons furthest from the nucleus, known as valence electrons, are crucial for determining a material's electrical properties. These outer electrons are loosely bound to the atom and are relatively free to move.
In conductors, such as metals like copper and silver, valence electrons are delocalized. This means they are not tightly bound to individual atoms but rather form a "sea" of electrons that can move freely throughout the material. When an electric field is applied, these electrons readily respond, creating an electric current. The abundance of free electrons and their high mobility are the hallmarks of good electrical conductivity.
Insulators, on the other hand, exhibit a starkly different behavior. Their valence electrons are tightly bound to their respective atoms. These electrons are not free to roam throughout the material. Strong electrostatic forces hold them firmly in place, preventing their movement under the influence of an electric field. This lack of mobile charge carriers is the primary reason why insulators resist the flow of electricity.
Band Theory: Visualizing Electron Energy Levels
To further understand the distinction between conductors and insulators, we need to consider band theory. This theory describes the energy levels of electrons in a solid material. Electrons can only exist at specific energy levels, and these levels are grouped into bands.
In a conductor, the valence band (the highest occupied energy band) overlaps with the conduction band (the band above the valence band). This overlap allows electrons from the valence band to easily transition to the conduction band, where they become free to move and contribute to the electric current. This essentially creates a continuous path for electron flow.
Insulators, however, exhibit a significant energy gap between the valence band and the conduction band. This gap, known as the band gap, represents a forbidden energy range for electrons. The energy required for an electron to jump from the valence band to the conduction band is substantial. At room temperature, the thermal energy available to electrons is insufficient to overcome this energy barrier. Therefore, very few electrons can transition to the conduction band, resulting in extremely low electrical conductivity.
The Size of the Band Gap: A Defining Factor
The size of the band gap is a crucial factor determining the insulating properties of a material. Larger band gaps correspond to better insulation. Materials with very large band gaps effectively trap their electrons, preventing any significant current flow. Conversely, materials with smaller band gaps, or those with overlapping valence and conduction bands, tend to be better conductors.
Factors Affecting Insulator Performance
While the fundamental principle of tightly bound electrons explains the basic insulating behavior, several other factors influence the effectiveness of an insulator in preventing current flow:
Temperature: Thermal Excitation
At higher temperatures, electrons gain more thermal energy. This increased energy can help some electrons overcome the band gap in insulators, leading to a slight increase in conductivity. However, this increase is generally small, and insulators remain highly resistive even at elevated temperatures.
Impurities and Defects: Weak Points in the Insulator
The presence of impurities or structural defects in an insulator can significantly affect its performance. These imperfections can create localized energy levels within the band gap, providing pathways for electrons to bypass the usual energy barrier. This can lead to increased conductivity, particularly at higher voltages where the electric field can assist electron movement through these defects. High-purity insulators are therefore essential for maintaining low conductivity.
Electric Field Strength: Breakdown Voltage
When a sufficiently high electric field is applied across an insulator, the electric force can overcome the binding energy of electrons, leading to dielectric breakdown. At this point, the insulator temporarily loses its insulating properties, and a large current can flow. The voltage at which dielectric breakdown occurs is called the breakdown voltage, and it is a critical parameter in insulator selection. Different insulators possess different breakdown voltages, highlighting the importance of choosing an appropriate insulator for a specific application. Understanding breakdown voltage is crucial in designing electrical systems to prevent short circuits and other failures.
Material Composition and Structure: Tailor-Made Insulators
The choice of material significantly impacts an insulator's performance. Different materials have different band gaps and are susceptible to various environmental factors. For example, some insulators are more resistant to moisture than others, which is a crucial consideration in outdoor applications. The structural arrangement of atoms within the material also influences its insulating capability. Crystalline insulators, with their highly ordered structure, often exhibit superior insulating properties compared to amorphous insulators, which have a more disordered atomic arrangement.
Examples of Common Insulators and Their Applications
Numerous materials serve as excellent insulators, each with specific properties suited for various applications:
-
Rubber: Widely used in electrical insulation due to its flexibility, durability, and good insulating properties. Used in wires, cables, and protective coverings.
-
Plastics (e.g., PVC, Teflon): Excellent insulators, often chosen for their low cost, ease of molding, and chemical resistance. Used extensively in electrical components and housings.
-
Glass: An excellent insulator used in high-voltage applications due to its high dielectric strength. Commonly found in electrical insulators and components.
-
Ceramics: High-temperature insulators with excellent dielectric properties. Used in high-temperature applications and high-voltage equipment.
-
Air: Acts as a natural insulator, separating conductors in many electrical systems. Plays a vital role in preventing unintended current flow.
The selection of an insulator depends critically on the specific application requirements. Factors such as operating temperature, voltage level, environmental conditions, and cost considerations guide the material choice.
Conclusion: Understanding Insulators is Crucial
Understanding why insulators do not conduct electricity is crucial for the design and operation of safe and reliable electrical systems. The inherent lack of free electrons, the presence of a significant band gap, and other material properties contribute to the superior insulating capabilities of these materials. By carefully considering the factors discussed in this article, engineers and scientists can select appropriate insulators for various applications, ensuring the efficient and safe operation of electrical equipment. The ability to manipulate and control electron movement in materials remains a cornerstone of modern technology, emphasizing the continued importance of research in this field. Future advancements in material science will undoubtedly lead to even more efficient and effective insulators, further enabling technological innovations.
Latest Posts
Latest Posts
-
How Many Sides Does A Polygon
Apr 24, 2025
-
How Do You Find The Center Of A Triangle
Apr 24, 2025
-
Which Of The Following Are Methods Used To Identify Bacteria
Apr 24, 2025
-
Moment Of Inertia Of A Thin Rod
Apr 24, 2025
-
Prime Minister Of India Age Limit
Apr 24, 2025
Related Post
Thank you for visiting our website which covers about Why Do Insulators Not Conduct Electricity . We hope the information provided has been useful to you. Feel free to contact us if you have any questions or need further assistance. See you next time and don't miss to bookmark.