Which Of The Following Is True Of Axons
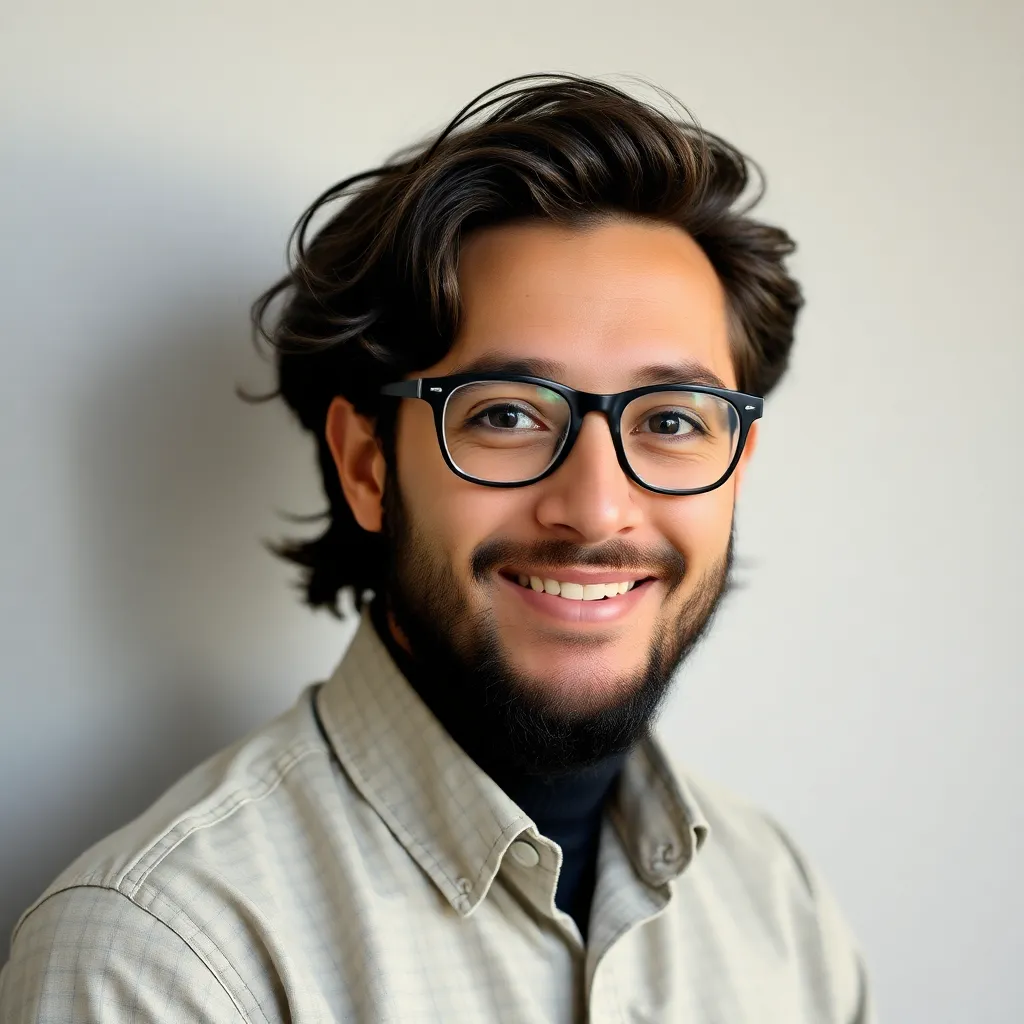
Juapaving
May 09, 2025 · 6 min read
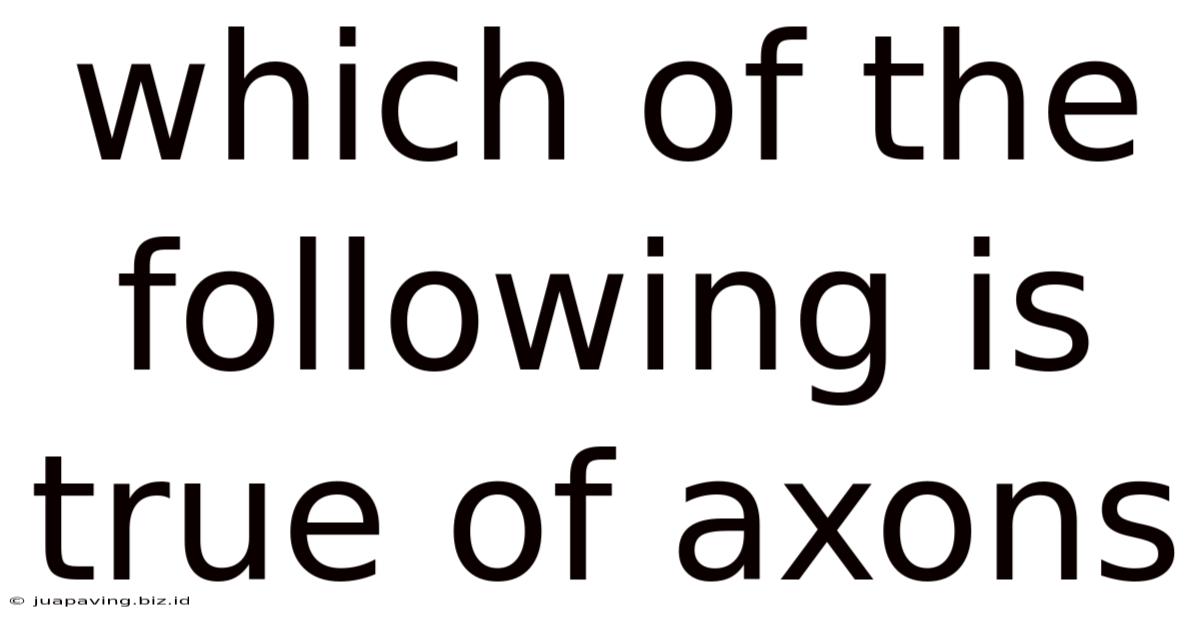
Table of Contents
Which of the Following is True of Axons? A Deep Dive into Neuronal Transmission
Understanding axons is crucial to comprehending the intricacies of the nervous system. These long, slender projections of neurons are the primary conduits for transmitting information throughout the body. This article will delve into the key characteristics of axons, addressing common questions and misconceptions surrounding their structure and function. We'll explore their role in neuronal communication, examining the processes of action potential generation and propagation, and highlighting their importance in various neurological functions.
The Fundamental Role of Axons in Neuronal Communication
Axons are essential components of neurons, specialized for the rapid transmission of electrical signals known as action potentials. These signals carry information from one neuron to another, enabling communication across the vast network of the nervous system. Unlike dendrites, which primarily receive signals, axons are designed for efficient signal transmission. This unidirectional flow of information is a defining characteristic of axonal function.
Key Characteristics of Axons:
-
Structure: Axons are typically long, thin cylindrical projections extending from the neuron's cell body (soma). Their length can vary dramatically, from a few micrometers to over a meter in some cases, such as those connecting the spinal cord to the toes. This remarkable length allows for signal transmission over significant distances within the body.
-
Myelin Sheath: Many axons are insulated by a myelin sheath, a fatty substance produced by glial cells (oligodendrocytes in the central nervous system and Schwann cells in the peripheral nervous system). This myelin sheath acts as an insulator, significantly increasing the speed of action potential conduction. The gaps between the myelin segments, known as Nodes of Ranvier, play a vital role in saltatory conduction, a process that accelerates signal transmission.
-
Axon Terminals (Synaptic Terminals): At their ends, axons branch into numerous axon terminals, also called synaptic terminals or boutons. These terminals form specialized junctions called synapses with other neurons or target cells (e.g., muscle cells, gland cells). At the synapse, the action potential triggers the release of neurotransmitters, chemical messengers that transmit the signal to the postsynaptic cell.
-
Axon Hillock: The axon hillock is the region where the axon originates from the neuron's soma. This crucial area is the site of action potential initiation. The summation of excitatory and inhibitory postsynaptic potentials (EPSPs and IPSPs) determines whether an action potential will be generated and propagated down the axon.
Action Potential Generation and Propagation: The Axon's Crucial Role
The process of action potential generation and propagation is intricately linked to the unique properties of the axon. Several factors contribute to this crucial process:
1. Resting Membrane Potential:
The axon, like the rest of the neuron, maintains a resting membrane potential—a voltage difference across its membrane. This potential is primarily due to the unequal distribution of ions (sodium, potassium, chloride) across the membrane, maintained by ion pumps and channels. This resting potential is typically around -70 mV.
2. Depolarization:
When a sufficient excitatory stimulus reaches the axon hillock, it triggers depolarization. Depolarization is a decrease in the membrane potential, making it less negative. If the depolarization reaches a threshold potential (typically around -55 mV), it triggers the opening of voltage-gated sodium channels.
3. Sodium Influx and Rising Phase:
The opening of voltage-gated sodium channels allows a rapid influx of sodium ions into the axon, causing a dramatic increase in membrane potential. This rapid depolarization constitutes the rising phase of the action potential.
4. Repolarization:
Following the peak of the action potential, voltage-gated potassium channels open, allowing potassium ions to flow out of the axon. This outflow of positive charge repolarizes the membrane, restoring it towards its resting potential.
5. Hyperpolarization:
The potassium channels often remain open slightly longer than needed, causing a temporary hyperpolarization—a membrane potential more negative than the resting potential. This ensures that the neuron is less likely to fire another action potential immediately.
6. Saltatory Conduction:
In myelinated axons, the action potential doesn't travel continuously down the axon. Instead, it jumps between the Nodes of Ranvier in a process called saltatory conduction. This "jumping" significantly increases the speed of conduction, allowing for rapid transmission of signals over long distances.
Axonal Transport: Maintaining the Integrity of the Axon
Axons are remarkably long and require efficient mechanisms to transport essential materials between the soma and the axon terminals. This process is known as axonal transport. There are two main types of axonal transport:
-
Anterograde transport: This moves materials from the soma towards the axon terminals. It's crucial for supplying the axon terminals with proteins, neurotransmitters, and other essential components necessary for synaptic transmission. Kinesin motor proteins play a vital role in this process.
-
Retrograde transport: This moves materials from the axon terminals back towards the soma. It’s important for recycling materials, signaling to the soma about the condition of the axon terminals, and transporting certain viruses or toxins back to the cell body. Dynein motor proteins are involved in retrograde transport.
Dysfunction in axonal transport can have serious consequences, contributing to various neurological disorders.
Axons and Neurological Disorders: The Implications of Axonal Damage
Damage to axons can have significant implications, leading to a range of neurological disorders. Several factors can contribute to axonal injury, including:
-
Trauma: Physical injuries, such as those resulting from accidents or surgery, can directly damage axons.
-
Ischemic injury: Lack of blood flow to the brain (stroke) can deprive axons of oxygen and nutrients, leading to damage.
-
Neurodegenerative diseases: In diseases like Alzheimer's and Parkinson's, axonal degeneration plays a significant role in the progression of the disease.
-
Multiple sclerosis (MS): This autoimmune disease targets the myelin sheath, resulting in impaired action potential conduction and neurological deficits.
-
Guillain-Barré syndrome: This autoimmune disorder affects the peripheral nervous system, leading to axonal demyelination and paralysis.
Axonal damage can lead to a range of symptoms, including muscle weakness, sensory loss, impaired coordination, and cognitive deficits, depending on the location and extent of the injury.
Axonal Regeneration: The Body's Capacity for Repair
While axons are generally considered incapable of regeneration in the central nervous system (CNS), there is a capacity for some degree of repair in the peripheral nervous system (PNS). Following injury, Schwann cells in the PNS play a crucial role in guiding axonal regrowth. However, regeneration in the CNS is limited due to the inhibitory environment created by glial scar formation and other factors.
Research is actively exploring strategies to promote axonal regeneration in the CNS, with promising avenues focusing on:
-
Inhibitory molecule neutralization: Blocking inhibitory molecules that prevent axonal regrowth in the CNS.
-
Growth factor stimulation: Introducing growth factors that stimulate axonal regeneration.
-
Cell transplantation: Transplanting cells that promote axonal growth.
Progress in this area could revolutionize the treatment of neurological disorders caused by axonal damage.
Conclusion: The Significance of Axons in Neural Function
Axons are fundamental components of the nervous system, responsible for transmitting information throughout the body. Their unique structure, including the myelin sheath and nodes of Ranvier, enables rapid and efficient action potential conduction. Understanding the intricacies of axonal function, action potential generation and propagation, and the consequences of axonal damage is paramount to advancing our understanding of neurological function and disease. Continued research into axonal regeneration holds immense promise for treating a wide range of neurological conditions. The exploration of axonal transport mechanisms also provides critical insights into maintaining the health and integrity of these essential neuronal structures. This detailed understanding of axons, from their basic structure and function to their involvement in neurological disorders, underpins the advancement of neurological research and the development of effective therapeutic interventions.
Latest Posts
Latest Posts
-
What State Of Matter Has No Definite Shape Or Volume
May 11, 2025
-
Least Common Multiple Of 54 And 72
May 11, 2025
-
Can Convex Mirrors Produce Real Images
May 11, 2025
-
Find The Current In 3 Ohm Resistor
May 11, 2025
-
What Does The Round Window In The Ear Do
May 11, 2025
Related Post
Thank you for visiting our website which covers about Which Of The Following Is True Of Axons . We hope the information provided has been useful to you. Feel free to contact us if you have any questions or need further assistance. See you next time and don't miss to bookmark.