What Organism Can Make Its Own Food
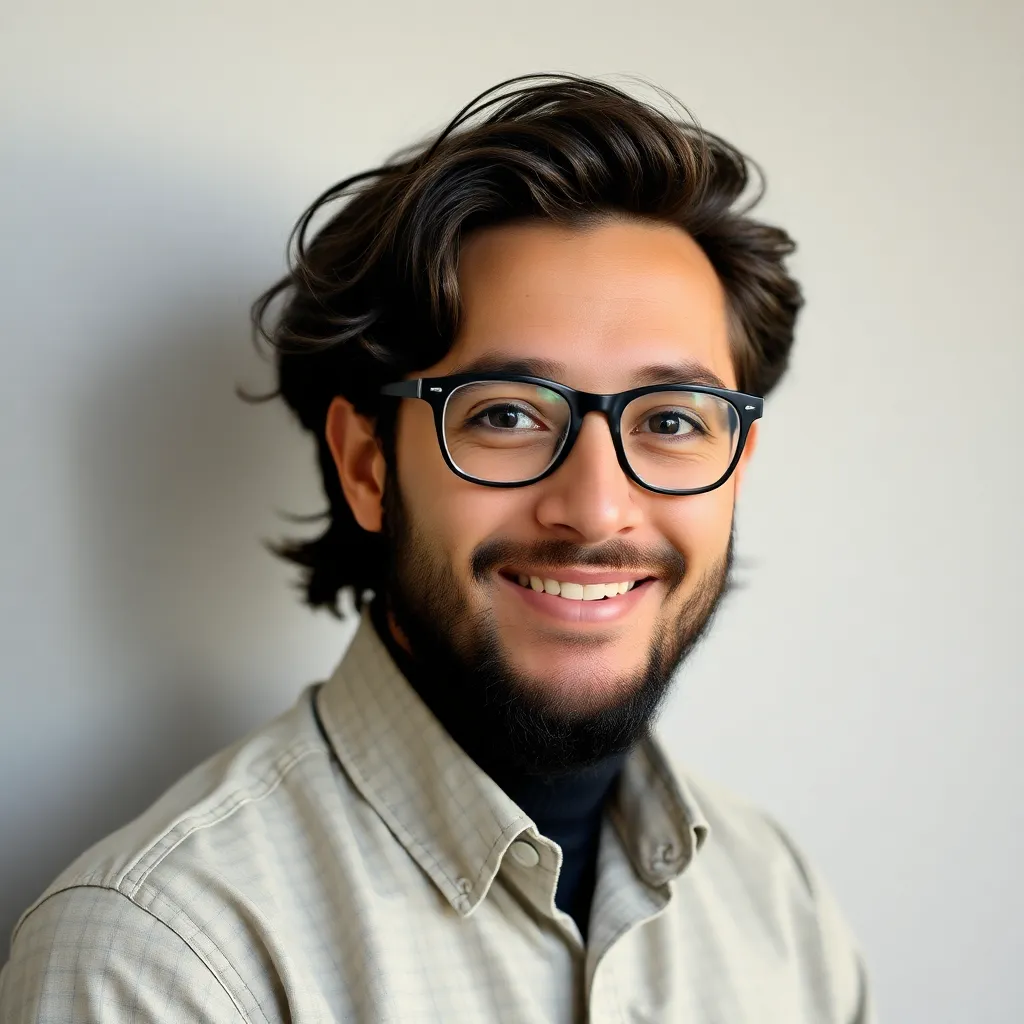
Juapaving
May 10, 2025 · 6 min read
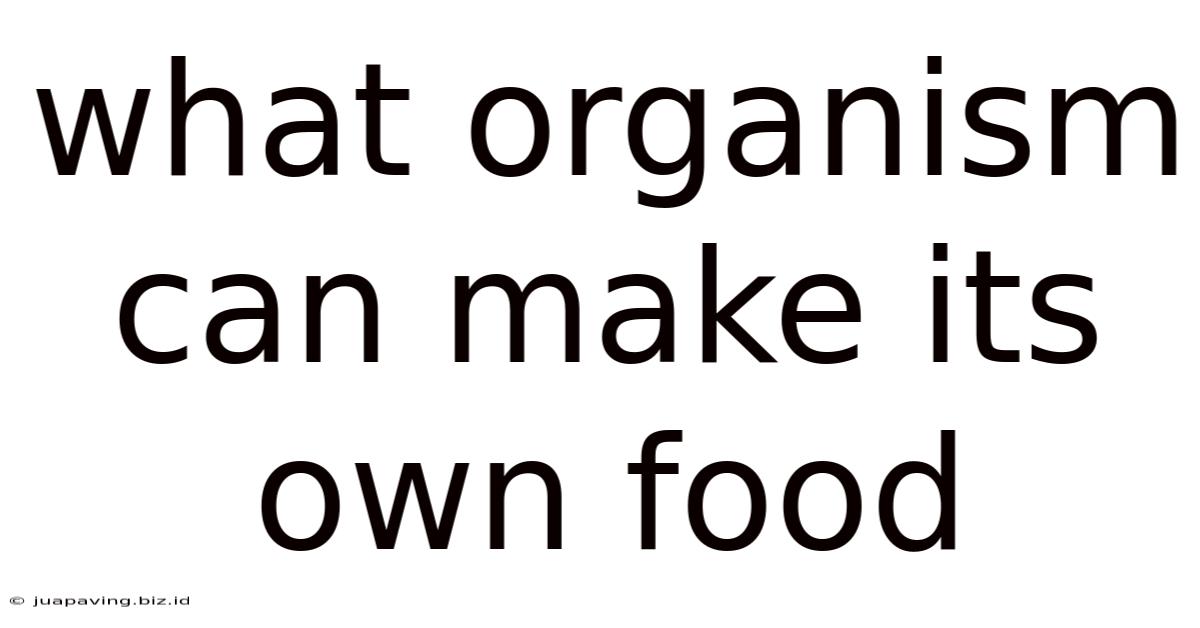
Table of Contents
What Organisms Can Make Their Own Food? A Deep Dive into Autotrophy
The ability to produce one's own food is a fundamental distinction in the biological world, separating organisms into two broad categories: autotrophs and heterotrophs. While heterotrophs rely on consuming other organisms for energy and nutrients, autotrophs, also known as producers, are capable of synthesizing their own organic compounds from inorganic sources. This remarkable feat underpins the entire food web, providing the foundational energy for all other life forms. This article will explore the fascinating world of autotrophy, examining the diverse mechanisms employed by various organisms to create their sustenance, the environmental significance of their processes, and the implications for the broader ecosystem.
The Power of Photosynthesis: The Sun's Energy Harvesters
The most widely known form of autotrophy is photosynthesis, a process that utilizes sunlight as the primary energy source. Photosynthetic organisms, primarily plants, algae, and cyanobacteria, contain specialized pigments, most notably chlorophyll, which capture light energy. This energy drives a complex series of biochemical reactions that convert carbon dioxide (CO2) and water (H2O) into glucose (C6H12O6), a simple sugar that serves as the building block for all other organic molecules. Oxygen (O2) is released as a byproduct.
The Photosynthesis Equation: A Simple Overview
The overall equation for photosynthesis succinctly summarizes this incredible transformation:
6CO₂ + 6H₂O + Light Energy → C₆H₁₂O₆ + 6O₂
This deceptively simple equation hides a multitude of intricate steps, involving several enzyme-catalyzed reactions within chloroplasts, the specialized organelles responsible for photosynthesis in plants and algae.
Variations on a Theme: Different Photosynthetic Pigments and Adaptations
While chlorophyll is the dominant pigment, many photosynthetic organisms utilize other pigments, such as carotenoids and phycobilins, to broaden their light-harvesting capabilities. This allows them to thrive in diverse light environments, from sun-drenched meadows to the depths of the ocean. For instance, red algae, abundant in deeper waters where blue light penetrates most effectively, possess phycoerythrin, a pigment that absorbs blue light efficiently.
Furthermore, photosynthetic organisms have developed various adaptations to optimize photosynthesis in different environments. CAM plants, such as cacti and succulents, adapted to arid climates, open their stomata (pores) at night to minimize water loss and fix carbon dioxide into organic acids, which are then used during the day for photosynthesis. C4 plants, like corn and sugarcane, utilize a specialized mechanism to concentrate carbon dioxide around the enzyme RuBisCO, enhancing photosynthetic efficiency in hot, dry conditions.
Beyond Sunlight: Chemosynthesis – Energy from Chemical Reactions
While photosynthesis relies on solar energy, chemosynthesis is a remarkable process where certain organisms utilize the energy released from chemical reactions to synthesize organic compounds. These organisms, primarily archaea and bacteria, are found in environments devoid of sunlight, such as deep-sea hydrothermal vents, volcanic hot springs, and even within rocks deep beneath the Earth’s surface.
Harnessing Chemical Energy: A Diverse Array of Electron Donors
Instead of sunlight, chemosynthetic organisms tap into the energy stored in inorganic chemical compounds. Different chemosynthetic organisms utilize various electron donors, including:
- Hydrogen sulfide (H₂S): This is a common electron donor in hydrothermal vent ecosystems. Organisms like Thiobacillus oxidize hydrogen sulfide to sulfate, releasing energy that is used to fix carbon dioxide.
- Ammonia (NH₃): Ammonia-oxidizing bacteria play a crucial role in the nitrogen cycle, using ammonia as an electron donor to produce nitrite.
- Iron (Fe²⁺): Iron-oxidizing bacteria obtain energy by oxidizing ferrous iron (Fe²⁺) to ferric iron (Fe³⁺).
- Methane (CH₄): Methanotrophic archaea utilize methane as an electron donor, oxidizing it to carbon dioxide.
The Chemosynthetic Ecosystem: A World of Darkness and Wonder
Chemosynthetic communities, often thriving in extreme environments, represent a remarkable example of life's adaptability. These ecosystems are fueled by the energy released from chemical reactions at the interfaces between different geological layers or within hydrothermal vents, supporting unique and often highly specialized food webs. Giant tube worms, for instance, rely on symbiotic chemosynthetic bacteria living within their bodies for sustenance.
The Ecological Significance of Autotrophs
Autotrophs are the foundation of virtually all ecosystems. Through photosynthesis and chemosynthesis, they convert inorganic matter into organic molecules, making energy and nutrients available to the entire food web. This process is vital for:
- Oxygen Production: Photosynthetic organisms are the primary producers of oxygen in the atmosphere, making it essential for the respiration of most organisms.
- Carbon Cycling: Autotrophs play a crucial role in the global carbon cycle, absorbing atmospheric carbon dioxide during photosynthesis and incorporating it into organic molecules. This process helps regulate Earth's climate.
- Nutrient Cycling: Through their metabolic processes, autotrophs facilitate the cycling of essential nutrients like nitrogen and phosphorus, making them available to other organisms.
- Food Web Structure: Autotrophs form the base of most food webs, providing the energy source for herbivores, which are then consumed by carnivores.
The Impact of Environmental Factors on Autotrophs
The productivity and distribution of autotrophs are significantly influenced by various environmental factors, including:
- Light Availability: Photosynthetic organisms require sufficient light for photosynthesis. Light intensity, duration, and spectral quality (wavelengths) all influence their growth and productivity.
- Nutrient Availability: The availability of essential nutrients, such as nitrogen, phosphorus, and other trace elements, limits autotrophic growth in many ecosystems.
- Temperature: Temperature affects the rate of enzyme-catalyzed reactions involved in photosynthesis and chemosynthesis. Extreme temperatures can inhibit or even halt these processes.
- Water Availability: Water is essential for photosynthesis and influences the availability of nutrients to autotrophs.
- Carbon Dioxide Concentration: Atmospheric carbon dioxide levels directly affect the rate of photosynthesis. Increased carbon dioxide levels can stimulate plant growth in some cases, although this effect is complex and can be influenced by other environmental factors.
Human Impact and the Future of Autotrophs
Human activities are significantly impacting autotrophic organisms and their vital roles in the environment. Deforestation, pollution, climate change, and habitat destruction are causing widespread declines in autotrophic populations and altering ecosystem functioning. These impacts have far-reaching consequences for biodiversity, food security, and climate regulation.
Conservation and Sustainability: Protecting Our Producers
Protecting autotrophic biodiversity and ensuring the continued functioning of ecosystems requires a multi-pronged approach, including:
- Protecting and restoring habitats: Conserving forests, wetlands, and other ecosystems crucial for autotrophic diversity is essential.
- Reducing pollution: Minimizing the release of pollutants into the environment is crucial to protect autotrophs from harmful effects.
- Mitigating climate change: Reducing greenhouse gas emissions is paramount to mitigating the negative impacts of climate change on autotrophs and their ecosystems.
- Sustainable agriculture practices: Promoting sustainable agricultural practices can enhance the health and productivity of autotrophic communities in agricultural lands.
- Promoting research and understanding: Increased research into the biology, ecology, and evolution of autotrophs is crucial for effective conservation and management strategies.
In conclusion, autotrophs, through their remarkable ability to synthesize their own food, are the cornerstone of life on Earth. Their processes, ranging from the ubiquitous photosynthesis to the remarkable chemosynthesis, are essential for maintaining the structure and function of ecosystems worldwide. Understanding the intricacies of autotrophy, its ecological significance, and the threats it faces is vital for ensuring the health of our planet and the well-being of future generations. Conserving and sustainably managing these vital organisms is not only environmentally responsible but also essential for maintaining the very foundations of life as we know it.
Latest Posts
Latest Posts
-
What Is 2 Root 2 An Example Of
May 10, 2025
-
Differentiate Between Plasma Membrane And Cell Membrane
May 10, 2025
-
What Is Ultimate Source Of Energy For Plants
May 10, 2025
-
5 Letter Word Beginning With Ap
May 10, 2025
-
In Photosynthesis The Oxygen Atoms In H2o Are
May 10, 2025
Related Post
Thank you for visiting our website which covers about What Organism Can Make Its Own Food . We hope the information provided has been useful to you. Feel free to contact us if you have any questions or need further assistance. See you next time and don't miss to bookmark.