What Is The Unit For Time In Physics
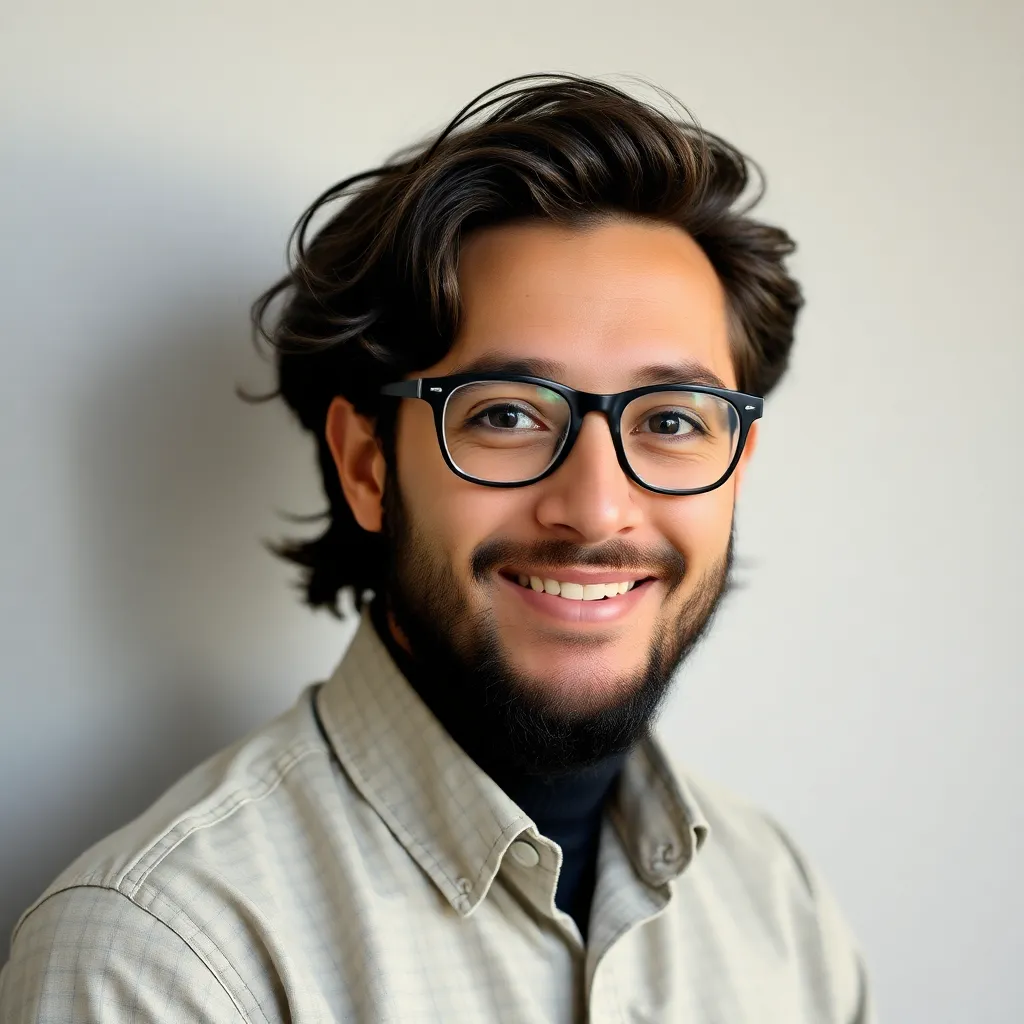
Juapaving
Apr 02, 2025 · 6 min read
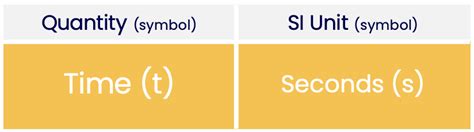
Table of Contents
- What Is The Unit For Time In Physics
- Table of Contents
- What is the Unit for Time in Physics? A Deep Dive into Temporal Measurement
- The Second: The Foundation of Temporal Measurement
- Historical Evolution of the Second's Definition
- The Modern Definition: Atomic Precision
- Applications of the Second in Physics and Beyond
- 1. Astronomy and Astrophysics: Measuring Cosmic Events
- 2. Particle Physics: Investigating the Subatomic World
- 3. Quantum Mechanics: Time-Dependent Phenomena
- 4. Nuclear Physics: Radioactive Decay Rates
- 5. GPS and Navigation Systems: Precise Time Synchronization
- 6. Engineering and Technology: Synchronization and Control
- Beyond the Second: Other Units of Time in Physics
- Future of Time Measurement: Pushing the Limits of Precision
- Conclusion: The Enduring Significance of the Second
- Latest Posts
- Latest Posts
- Related Post
What is the Unit for Time in Physics? A Deep Dive into Temporal Measurement
The seemingly simple question, "What is the unit for time in physics?" opens a fascinating door into the intricate world of measurement, fundamental constants, and the very fabric of spacetime. While the answer at a basic level is the second, a deeper exploration reveals layers of complexity, historical context, and ongoing scientific inquiry. This article delves into the definition, history, and applications of the unit of time in physics, examining its role in various fields and the ongoing efforts to refine its definition.
The Second: The Foundation of Temporal Measurement
The second (s) is the fundamental unit of time in the International System of Units (SI), the globally accepted standard for measurement. For many everyday applications, this simple definition suffices. However, the precise definition of the second has evolved significantly throughout history, reflecting advancements in our understanding of physics and technology.
Historical Evolution of the Second's Definition
Initially, the second was defined as a fraction of a day – specifically, 1/86400 of a mean solar day (the average time it takes the Earth to rotate once on its axis). This definition, while practical for centuries, suffered from inherent limitations. The Earth's rotation isn't perfectly uniform; its speed varies slightly due to factors like tidal forces and the shifting of Earth's mass distribution. This variability made the solar day an imprecise basis for defining a fundamental unit.
The need for a more stable and precise definition led to a shift in the 20th century. Atomic clocks, based on the precise and consistent oscillations of atoms, provided a far more accurate timekeeping mechanism. Consequently, the definition of the second was redefined in 1967 using atomic transitions.
The Modern Definition: Atomic Precision
Currently, the second is defined as: "the duration of 9,192,631,770 periods of the radiation corresponding to the transition between the two hyperfine levels of the ground state of the caesium-133 atom."
This definition relies on the incredibly precise and stable oscillations of caesium-133 atoms. These oscillations are virtually unaffected by external factors, providing a far more consistent and accurate standard than the Earth's rotation. This atomic definition has dramatically improved the accuracy of timekeeping, crucial for numerous scientific and technological applications.
Applications of the Second in Physics and Beyond
The second, as the fundamental unit of time, plays a vital role across a vast range of scientific disciplines and technological applications. Its precise measurement is fundamental to our understanding of the universe and the development of advanced technologies.
1. Astronomy and Astrophysics: Measuring Cosmic Events
In astronomy and astrophysics, the second is essential for measuring the vast timescales involved in celestial events. From the orbital periods of planets and stars to the lifespan of galaxies and the expansion of the universe, accurate timekeeping is paramount. Precise timing is crucial in analyzing data from telescopes, coordinating observations across different instruments, and understanding the dynamics of celestial bodies. Cosmological models, for example, heavily rely on precise measurements of time to determine parameters like the Hubble constant and the age of the universe.
2. Particle Physics: Investigating the Subatomic World
In particle physics, the second, or rather, incredibly small fractions of a second (like picoseconds or femtoseconds), becomes crucial. Experiments at particle accelerators, like the Large Hadron Collider (LHC), involve measuring incredibly short-lived particles with lifetimes measured in fractions of a second. These measurements are essential for understanding the fundamental forces and particles that govern the universe at the most fundamental level. High-precision timing systems are essential for coordinating the actions of detectors and analyzing the results of these experiments.
3. Quantum Mechanics: Time-Dependent Phenomena
Quantum mechanics, the theory governing the behavior of matter at the atomic and subatomic level, is intrinsically time-dependent. Many quantum phenomena, like radioactive decay or the evolution of wave functions, unfold over specific timescales. Accurate measurement of time is crucial for testing the predictions of quantum mechanics and understanding the behavior of quantum systems. For example, the study of quantum entanglement and quantum coherence often relies on precise timing measurements to establish the correlations between quantum systems.
4. Nuclear Physics: Radioactive Decay Rates
In nuclear physics, the concept of half-life – the time it takes for half of a radioactive substance to decay – directly involves the second as a unit. This property is critical in various applications, from nuclear medicine and dating techniques (like carbon dating) to understanding nuclear reactions in stars. The accurate measurement of decay rates is essential for understanding the stability of atomic nuclei and the properties of radioactive isotopes.
5. GPS and Navigation Systems: Precise Time Synchronization
Global Positioning Systems (GPS) rely heavily on extremely precise time synchronization. GPS satellites contain atomic clocks that transmit signals to receivers on Earth. By measuring the time it takes for these signals to travel, the receiver can determine its precise location. The accuracy of GPS technology is directly dependent on the accuracy of the atomic clocks and the precise measurement of time. Even small errors in time measurement can lead to significant errors in location determination.
6. Engineering and Technology: Synchronization and Control
Precise timing is crucial in numerous engineering and technological applications. In telecommunications, synchronization of signals is essential for effective communication. In high-speed digital electronics, the timing of signals is crucial for proper functioning. Furthermore, industrial control systems often rely on precise timing mechanisms to coordinate the operations of various components.
Beyond the Second: Other Units of Time in Physics
While the second is the fundamental unit, other units derived from it are used extensively in physics, depending on the specific timescale involved:
- Millisecond (ms): 1/1000 of a second
- Microsecond (µs): 1/1,000,000 of a second
- Nanosecond (ns): 1/1,000,000,000 of a second
- Picosecond (ps): 1/1,000,000,000,000 of a second
- Femtosecond (fs): 1/1,000,000,000,000,000 of a second
- Minute (min): 60 seconds
- Hour (h): 3600 seconds
- Day: 86400 seconds
- Year: Approximately 31,536,000 seconds (varies slightly depending on whether it's a leap year)
The choice of unit depends on the specific application and the timescale involved. For cosmological phenomena, years or even billions of years might be appropriate. Conversely, for processes in particle physics, femtoseconds or even attoseconds might be needed.
Future of Time Measurement: Pushing the Limits of Precision
Research continues to improve the accuracy and precision of time measurement. Scientists are exploring new atomic clocks based on different elements and techniques, pushing the limits of what's achievable. This ongoing quest for ever-higher precision has implications for fundamental physics, such as testing theories of general relativity and improving our understanding of the universe.
Optical clocks, for example, use optical transitions in atoms, potentially offering even greater precision than current caesium-based clocks. The development of such technologies could lead to even more accurate GPS systems, more precise experiments in particle physics, and a deeper understanding of fundamental physical constants.
Conclusion: The Enduring Significance of the Second
The seemingly simple unit of the second holds profound significance in physics and beyond. From its humble beginnings as a fraction of a day to its current definition based on the incredibly precise oscillations of caesium atoms, the second has undergone a remarkable evolution. Its precise measurement is essential for numerous scientific disciplines and technological applications, driving advancements in our understanding of the universe and the development of innovative technologies. As research continues to push the boundaries of time measurement, the role of the second in shaping our knowledge and technology will only grow in importance.
Latest Posts
Latest Posts
-
How Many Feet Is 26 Inches
Apr 05, 2025
-
Actin And Myosin Are What Type Of Biological Molecule
Apr 05, 2025
-
Fluid Part Of Blood After Removal Of Corpuscles Is
Apr 05, 2025
-
Is A Substance In Which Another Substance Is Dissolved
Apr 05, 2025
-
Empirical Formula Calculator For Ionic Compounds
Apr 05, 2025
Related Post
Thank you for visiting our website which covers about What Is The Unit For Time In Physics . We hope the information provided has been useful to you. Feel free to contact us if you have any questions or need further assistance. See you next time and don't miss to bookmark.