What Is The Final Electron Acceptor In Aerobic Cellular Respiration
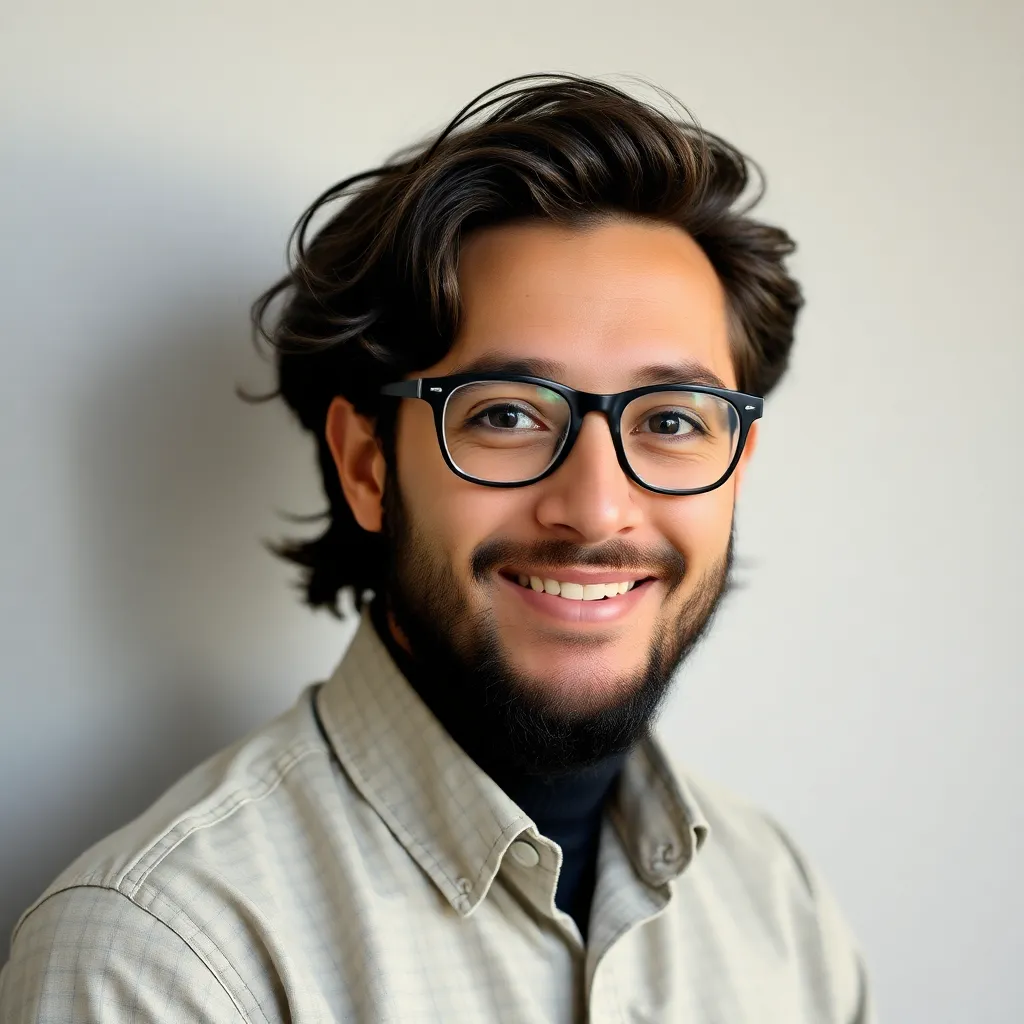
Juapaving
Apr 19, 2025 · 6 min read

Table of Contents
What is the Final Electron Acceptor in Aerobic Cellular Respiration?
Aerobic cellular respiration is the process by which cells break down glucose in the presence of oxygen to generate energy in the form of ATP (adenosine triphosphate). This intricate process involves a series of interconnected reactions, culminating in the production of a significant amount of ATP – the cell's primary energy currency. Understanding the intricacies of aerobic respiration is crucial to grasping the fundamental processes of life, and a key component of this understanding is identifying the final electron acceptor. This article will delve deep into this topic, exploring the role of the final electron acceptor, its significance in ATP production, and the consequences of its absence.
The Electron Transport Chain: A Cascade of Energy Transfer
Before we pinpoint the final electron acceptor, let's establish the context. Aerobic cellular respiration is broadly divided into four main stages: glycolysis, pyruvate oxidation, the Krebs cycle (also known as the citric acid cycle), and oxidative phosphorylation. It's within oxidative phosphorylation that the electron transport chain (ETC) operates, and this is where the final electron acceptor plays its crucial role.
The ETC is a series of protein complexes embedded within the inner mitochondrial membrane (in eukaryotes) or the plasma membrane (in prokaryotes). These complexes act as electron carriers, sequentially passing electrons down an energy gradient. This electron flow is coupled to the pumping of protons (H+) from the mitochondrial matrix (or cytoplasm) to the intermembrane space (or periplasm), creating a proton gradient. This gradient is a form of stored energy, crucial for the next stage.
The Role of Electron Carriers
Electrons don't magically appear in the ETC. They are derived from the breakdown of glucose during glycolysis, pyruvate oxidation, and the Krebs cycle. These initial stages generate high-energy electron carriers, namely NADH and FADH2. These molecules act as electron shuttles, carrying electrons from the earlier stages of respiration to the ETC. They deliver their high-energy electrons to the initial complexes of the electron transport chain.
Oxygen: The Ultimate Electron Acceptor
Finally, we arrive at the heart of the matter: the final electron acceptor in aerobic cellular respiration is oxygen (O2). This seemingly simple molecule plays a pivotal role in the entire process. As electrons travel down the ETC, they progressively lose energy. This energy is harnessed to pump protons across the membrane. At the end of the ETC, these electrons, now significantly low in energy, are transferred to oxygen molecules. This oxygen molecule accepts the electrons, and along with protons from the mitochondrial matrix, it is reduced to form water (H2O).
The equation summarizing this final step is:
4e- + 4H+ + O2 → 2H2O
The Significance of Oxygen's Role
Oxygen's role as the final electron acceptor is absolutely critical for the efficient generation of ATP. Without it, the electron transport chain would come to a halt. The electron carriers (NADH and FADH2) would become saturated with electrons, preventing further breakdown of glucose and drastically reducing ATP production. This is why aerobic respiration is so much more efficient than anaerobic respiration.
Chemiosmosis: Harnessing the Proton Gradient
The pumping of protons across the membrane during electron transport creates a proton gradient – a difference in proton concentration across the membrane. This gradient represents a store of potential energy. This energy is then harnessed by ATP synthase, an enzyme embedded in the inner mitochondrial membrane. Protons flow back down their concentration gradient, through ATP synthase, driving the synthesis of ATP from ADP and inorganic phosphate (Pi). This process is called chemiosmosis.
ATP Synthase: The ATP Production Powerhouse
ATP synthase is a remarkable molecular machine, acting as a rotary motor. The flow of protons through ATP synthase causes a rotation, which in turn drives the synthesis of ATP. This is a highly efficient process, producing a substantial amount of ATP molecules – the primary energy source for most cellular functions. The vast majority of ATP generated during cellular respiration is produced via chemiosmosis driven by the electron transport chain and the final reduction of oxygen.
Consequences of the Absence of Oxygen: Anaerobic Respiration
When oxygen is unavailable, the electron transport chain cannot function as described above. The electron carriers become saturated, and ATP production through oxidative phosphorylation grinds to a halt. Cells then resort to alternative mechanisms to generate ATP, collectively known as anaerobic respiration (or fermentation).
Anaerobic respiration uses alternative electron acceptors, such as sulfate (SO42-), nitrate (NO3-), or even organic molecules like pyruvate. However, these processes are significantly less efficient in ATP production than aerobic respiration. The absence of oxygen leads to a dramatic reduction in ATP yield.
Fermentation: A Less Efficient Pathway
Fermentation, a common form of anaerobic respiration, represents a pathway for regenerating NAD+ from NADH, thereby allowing glycolysis to continue. In this case, pyruvate acts as the electron acceptor. This produces either lactate (in lactic acid fermentation) or ethanol and carbon dioxide (in alcoholic fermentation). While fermentation allows for some ATP production through glycolysis, the net ATP yield is much lower compared to aerobic respiration. This explains the limited energy available to organisms relying on anaerobic metabolism.
The Evolutionary Significance of Oxygen as the Final Electron Acceptor
The evolution of oxygenic photosynthesis profoundly impacted life on Earth. The introduction of significant levels of oxygen into the atmosphere facilitated the evolution of aerobic respiration, a far more efficient process for energy generation than anaerobic pathways. The high ATP yield from aerobic respiration allowed for the development of complex multicellular organisms and the diversification of life as we know it. The evolution of oxygen as the final electron acceptor is a crucial step in the history of life.
Conclusion: Oxygen – The Key to Efficient Energy Production
In summary, oxygen serves as the final electron acceptor in aerobic cellular respiration, a role of paramount importance. Its acceptance of electrons at the end of the electron transport chain is essential for the establishment of the proton gradient, driving the chemiosmotic synthesis of ATP – the primary energy currency of the cell. Without oxygen, the efficiency of energy production plummets, as cells resort to less productive anaerobic pathways. Understanding the role of oxygen as the final electron acceptor is fundamental to understanding the intricacies of cellular respiration and the energy dynamics of life itself. This knowledge has far-reaching implications in fields like medicine, biotechnology, and environmental science. Furthermore, the study of alternative electron acceptors in anaerobic respiration continues to be a field of active research, uncovering new insights into the adaptability and diversity of life on Earth. The seemingly simple act of oxygen accepting electrons is a crucial process that underpins the very existence of complex life.
Latest Posts
Latest Posts
-
Things Beginning With The Letter N
Apr 20, 2025
-
Difference Between Starch And Cellulose And Glycogen
Apr 20, 2025
-
Convex Mirror Is Converging Or Diverging
Apr 20, 2025
-
Composite Numbers From 1 To 1000
Apr 20, 2025
-
Another Name For The Water Cycle
Apr 20, 2025
Related Post
Thank you for visiting our website which covers about What Is The Final Electron Acceptor In Aerobic Cellular Respiration . We hope the information provided has been useful to you. Feel free to contact us if you have any questions or need further assistance. See you next time and don't miss to bookmark.