What Is The Capacity To Do Work
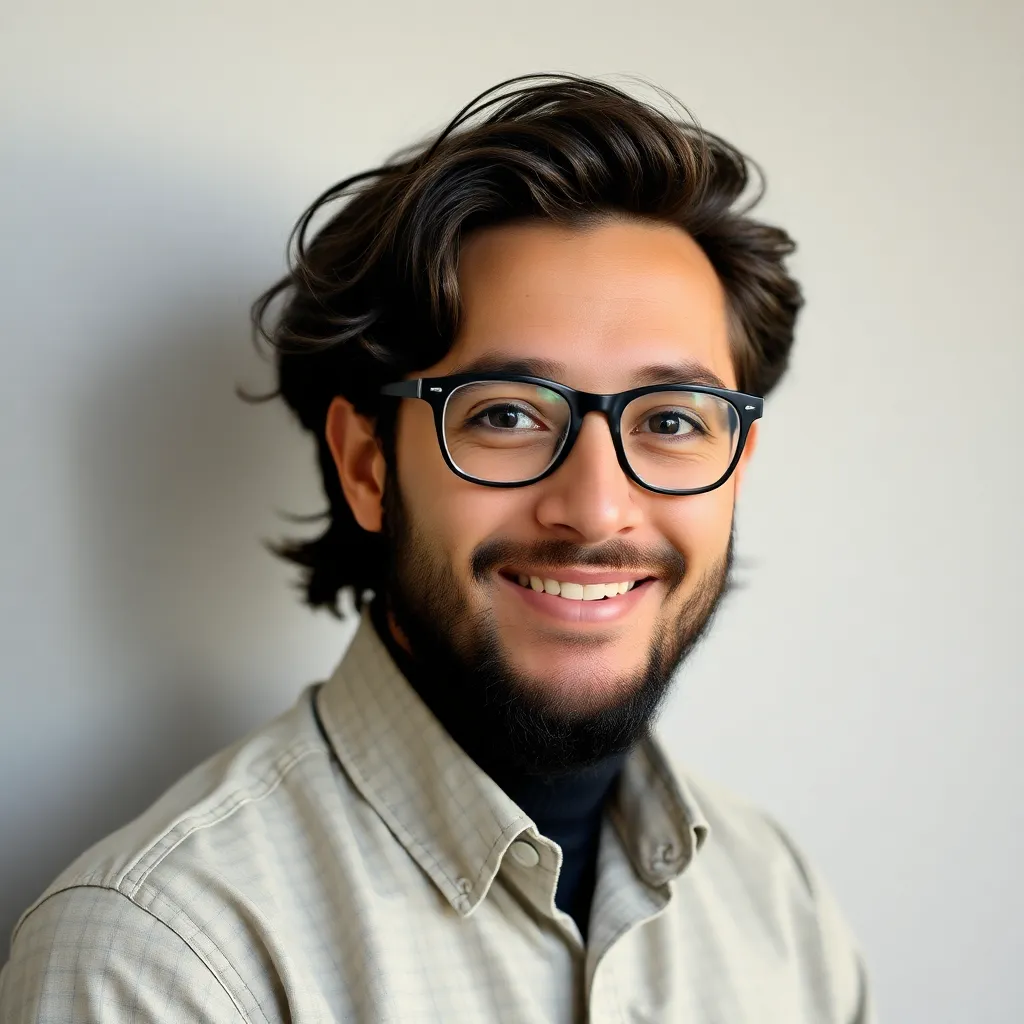
Juapaving
Apr 26, 2025 · 6 min read

Table of Contents
What is the Capacity to Do Work? A Deep Dive into Energy and Power
The capacity to do work is a fundamental concept in physics and engineering, encompassing the ability of a system to exert force over a distance, resulting in a change in its state or the state of its surroundings. While seemingly simple, this concept underlies a vast array of phenomena, from the microscopic workings of cells to the macroscopic forces shaping our universe. This article will explore the intricacies of this capacity, delving into the related concepts of energy and power, and examining their applications in diverse fields.
Understanding Work: Force and Displacement
At its core, the capacity to do work is intrinsically linked to the ability to perform work. In physics, work is defined as the product of force and displacement. Specifically, it's the force applied to an object multiplied by the distance the object moves in the direction of the force. Mathematically, this is represented as:
W = Fd cosθ
Where:
- W represents work
- F represents force
- d represents displacement
- θ represents the angle between the force and the displacement vector.
This equation highlights a crucial point: work is only done when there's a force acting on an object and that object moves in the direction of the force. Pushing against an immovable wall, for example, results in no work being done, despite the effort exerted. The angle θ clarifies that only the component of the force parallel to the displacement contributes to the work done.
Units of Work
The standard unit of work in the International System of Units (SI) is the joule (J). One joule is defined as the work done when a force of one newton is applied over a displacement of one meter. Other units, such as the erg (in the CGS system) and the foot-pound (in the imperial system), also exist but are less commonly used in scientific and engineering contexts.
Energy: The Capacity to Do Work
Energy is the cornerstone of the capacity to do work. It's defined as the capacity of a system to perform work. Energy exists in various forms, including:
-
Kinetic Energy: The energy possessed by an object due to its motion. It's directly proportional to the object's mass and the square of its velocity. Mathematically: KE = ½mv²
-
Potential Energy: The energy stored within an object due to its position or configuration. Examples include gravitational potential energy (related to height) and elastic potential energy (stored in a stretched spring).
-
Thermal Energy: The energy associated with the random motion of atoms and molecules within a substance. Temperature is a measure of the average kinetic energy of these particles.
-
Chemical Energy: Energy stored in the bonds between atoms and molecules. The release of chemical energy fuels many processes, including cellular respiration and combustion.
-
Nuclear Energy: Energy stored within the nucleus of an atom. Nuclear fission and fusion release enormous amounts of energy.
-
Radiant Energy: Energy transmitted as electromagnetic waves, including light, radio waves, and X-rays.
These different forms of energy are interconvertible. For instance, gravitational potential energy can be converted into kinetic energy as an object falls, or chemical energy can be converted into thermal energy through combustion. This interconversion is governed by the law of conservation of energy, which states that energy cannot be created or destroyed, only transformed from one form to another.
Units of Energy
Like work, the SI unit for energy is the joule (J). Other units, such as the calorie (cal) and the kilowatt-hour (kWh), are also frequently used, depending on the context.
Power: The Rate of Doing Work
Power is a measure of how quickly work is done or energy is transferred. It's defined as the rate at which work is performed or energy is consumed. Mathematically:
P = W/t
Where:
- P represents power
- W represents work
- t represents time
Alternatively, power can be expressed as the rate of energy transfer:
P = ΔE/t
Where:
- ΔE represents the change in energy.
Units of Power
The SI unit of power is the watt (W), defined as one joule per second (J/s). Other units, such as horsepower (hp) and kilowatts (kW), are also commonly used.
Applications of the Capacity to Do Work
The capacity to do work, as embodied by energy and power, is fundamental to countless aspects of our lives and the functioning of the universe. Consider the following examples:
-
Transportation: Vehicles, from cars to rockets, rely on the conversion of energy (chemical, electrical) into kinetic energy to achieve motion. The power of an engine determines its acceleration and speed capabilities.
-
Manufacturing: Industrial processes, such as machining and forging, utilize significant amounts of energy to shape materials. The efficiency of these processes is directly related to the power available.
-
Electricity Generation: Power plants convert various forms of energy (nuclear, thermal, hydroelectric) into electrical energy, which powers our homes and industries. The capacity of a power plant to generate electricity is measured in terms of its power output.
-
Biological Systems: Living organisms harness energy from their environment to perform work, from cellular processes to locomotion. Metabolic processes involve the conversion and transfer of energy to sustain life.
-
Communication: The transmission of information, whether through radio waves or optical fibers, relies on the transfer of energy in the form of electromagnetic radiation.
-
Climate Systems: Weather patterns and climate change are driven by the transfer and transformation of energy within the Earth's atmosphere and oceans. Solar radiation is a primary source of energy for these systems.
Efficiency and Losses
In any real-world system, the capacity to do work is never fully utilized. Energy losses always occur due to factors such as friction, heat transfer, and inefficiencies in energy conversion processes. Efficiency is a measure of how effectively a system converts one form of energy into another, typically expressed as a percentage:
Efficiency = (Useful energy output / Total energy input) x 100%
Improving efficiency is a crucial aspect of engineering design and technological advancement, as it reduces energy consumption and environmental impact.
Conclusion: The Ubiquitous Nature of Work Capacity
The capacity to do work, through its manifestation as energy and power, is a fundamental concept that pervades all aspects of the natural world and human civilization. From the smallest biological processes to the largest engineering feats, understanding this capacity is crucial for technological advancement, scientific discovery, and even our comprehension of the universe itself. Further exploration into the various forms of energy, their interconversion, and the efficiency of energy utilization will continue to drive innovation and shape our future. This fundamental principle underpins our modern world and continues to present exciting challenges and opportunities for research and development. The continuous pursuit of higher efficiency and the exploration of new energy sources are vital for a sustainable and technologically advanced future.
Latest Posts
Latest Posts
-
8 Letter Words Beginning With A
Apr 26, 2025
-
Common Multiples Of 9 And 10
Apr 26, 2025
-
How Many Chambers Of The Heart Do Amphibians Have
Apr 26, 2025
-
How Do You Calculate Rf Values Chromatography
Apr 26, 2025
-
33 1 3 To A Fraction
Apr 26, 2025
Related Post
Thank you for visiting our website which covers about What Is The Capacity To Do Work . We hope the information provided has been useful to you. Feel free to contact us if you have any questions or need further assistance. See you next time and don't miss to bookmark.