What Do Atoms Actually Look Like
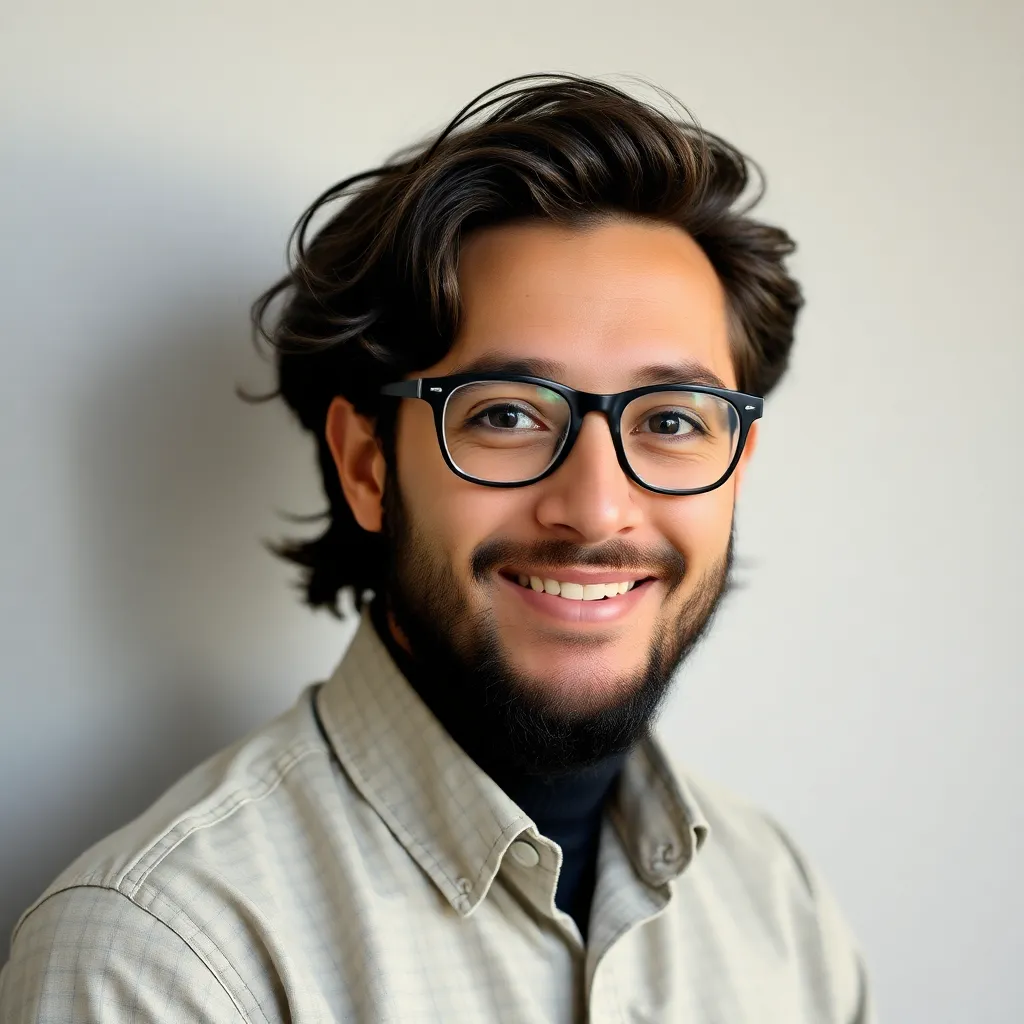
Juapaving
Apr 23, 2025 · 7 min read

Table of Contents
What Do Atoms Actually Look Like? A Deep Dive into the Subatomic World
The question, "What do atoms actually look like?", seems deceptively simple. After all, we've all seen those diagrams in textbooks – a central nucleus orbited by electrons like planets around a sun. But this image, while helpful for visualizing basic atomic structure, is a gross oversimplification. The reality is far more nuanced, complex, and frankly, mind-bending. This article will delve into the true nature of atoms, exploring their composition, behavior, and the limitations of our ability to truly "see" them.
The Limitations of Visualizing the Subatomic World
Before we dive into the details, it's crucial to understand a fundamental limitation: we can't directly "see" atoms in the way we see everyday objects. Visible light, which allows us to perceive the world around us, interacts with objects much larger than atoms. To "see" something, light needs to bounce off it and be detected by our eyes or instruments. Atoms, however, are far smaller than the wavelength of visible light. This means that light simply passes right through them without interacting significantly, making them invisible to conventional optical microscopes.
This limitation necessitates the use of other methods to investigate the atomic realm. Techniques like electron microscopy, scanning tunneling microscopy, and X-ray diffraction offer indirect ways to probe atomic structure, providing us with information about their arrangement and properties. However, even these advanced techniques provide us with models and interpretations rather than direct visual representations.
The Atomic Model: A Historical Perspective
Our understanding of the atom has evolved dramatically over time. Early models, like Dalton's solid sphere model, were rudimentary. J.J. Thomson's plum pudding model, proposing a positively charged sphere with embedded negatively charged electrons, was a significant step forward. However, it was Rutherford's gold foil experiment that revolutionized our understanding. By observing the scattering of alpha particles, Rutherford concluded that most of an atom's mass and positive charge are concentrated in a tiny, dense nucleus, with electrons orbiting it at a significant distance.
This nuclear model, while groundbreaking, still presented challenges. Classical physics predicted that orbiting electrons should constantly emit radiation, losing energy and spiraling into the nucleus, causing the atom to collapse. This paradox was resolved by the advent of quantum mechanics.
The Quantum Mechanical Model: Probability Clouds and Orbitals
The quantum mechanical model of the atom, developed in the early 20th century, fundamentally altered our understanding. It replaces the simplistic image of electrons orbiting the nucleus like planets with a far more probabilistic description. Electrons don't follow well-defined paths; instead, they exist in atomic orbitals, regions of space where the probability of finding an electron is high.
These orbitals are not rigid shapes like the orbits in the planetary model. Instead, they are fuzzy, three-dimensional probability clouds. The shape and energy level of an orbital depend on the quantum numbers associated with the electron's state. This means that electrons are not located at specific points but rather have a probability distribution across the orbital. The higher the probability, the more likely it is to find an electron in that region.
Understanding Quantum Numbers
Quantum numbers describe the various properties of an electron within an atom and determine the shape and energy of its orbital.
-
Principal quantum number (n): This number determines the electron's energy level and its average distance from the nucleus. Higher 'n' values correspond to higher energy levels and greater average distances.
-
Azimuthal quantum number (l): This number describes the shape of the electron's orbital. 'l' can range from 0 to n-1. 'l' = 0 corresponds to an s orbital (spherical), 'l' = 1 corresponds to p orbitals (dumbbell-shaped), 'l' = 2 corresponds to d orbitals (more complex shapes), and so on.
-
Magnetic quantum number (ml): This number specifies the orientation of the orbital in space. 'ml' can range from -l to +l. For example, a p orbital (l=1) can have three orientations (ml = -1, 0, +1), corresponding to px, py, and pz orbitals.
-
Spin quantum number (ms): This number describes the intrinsic angular momentum of the electron, often visualized as a spin on its axis. It can have two values: +1/2 (spin up) and -1/2 (spin down).
The Nucleus: Protons, Neutrons, and the Strong Force
The nucleus, the atom's core, is incredibly dense and contains nearly all of the atom's mass. It's composed of protons, which carry a positive charge, and neutrons, which are electrically neutral. The number of protons in the nucleus (the atomic number) determines the element. For example, an atom with one proton is hydrogen, while an atom with six protons is carbon. The number of neutrons can vary, leading to isotopes of the same element.
Holding the nucleus together against the repulsive forces between positively charged protons is the strong nuclear force, one of the four fundamental forces of nature. This force is much stronger than the electromagnetic force at short distances but has a very short range. The balance between the strong nuclear force and the electromagnetic repulsion determines the stability of the nucleus. Unstable nuclei undergo radioactive decay, emitting particles or energy to achieve a more stable configuration.
Visualizing Atoms: Techniques and Interpretations
As mentioned earlier, we can't directly "see" atoms. However, various techniques allow us to indirectly visualize their structure and behavior:
-
Electron Microscopy: This technique uses a beam of electrons to create images of surfaces. While it can't resolve individual atoms in all cases, it can provide images of atomic arrangements in certain materials.
-
Scanning Tunneling Microscopy (STM): STM uses a sharp tip to scan a surface, measuring the tunneling current between the tip and the surface. This allows for incredibly high-resolution images, capable of resolving individual atoms in some cases.
-
X-ray Diffraction: This technique uses X-rays to determine the arrangement of atoms in a crystal lattice. By analyzing the diffraction pattern, scientists can deduce the positions of atoms within the material.
It's important to remember that the images produced by these techniques are often interpretations of data rather than direct visual representations. They provide valuable insights into atomic structure but don't show atoms as we might visualize them in everyday life.
Beyond the Basics: Quantum Effects and Atomic Behavior
The quantum nature of atoms leads to a range of fascinating phenomena:
-
Quantum Tunneling: Electrons have a probability of passing through energy barriers, even if they don't have enough energy to overcome them classically. This phenomenon is crucial in many chemical reactions and technological applications.
-
Quantum Superposition: Before measurement, an electron can exist in multiple states simultaneously. This is not simply a lack of knowledge about the electron's state, but rather a fundamental property of quantum mechanics.
-
Quantum Entanglement: Two or more particles can become linked in such a way that their fates are intertwined, regardless of the distance separating them. Measuring the state of one particle instantaneously determines the state of the other.
Conclusion: The Elusive Image of an Atom
The question of what atoms actually look like is far more complex than a simple picture can convey. While the textbook model of a nucleus orbited by electrons serves as a useful starting point, the reality is far more intricate. Atoms are governed by the principles of quantum mechanics, exhibiting probabilistic behavior and defying classical intuition. Techniques like electron microscopy, STM, and X-ray diffraction provide indirect insights into atomic structure, but these are interpretations of data, not direct visual observations. The true image of an atom is a blend of probability clouds, quantum effects, and the powerful forces that govern the subatomic world – a testament to the profound and enigmatic nature of the universe at its most fundamental level. The quest to understand atoms is ongoing, pushing the boundaries of scientific inquiry and revealing the incredible complexity and beauty hidden within the seemingly simple building blocks of matter.
Latest Posts
Latest Posts
-
Burning Candle Physical Or Chemical Change
Apr 23, 2025
-
The Si Unit For Energy Is
Apr 23, 2025
-
Finding The Angle Between Two Planes
Apr 23, 2025
-
What Is The Colour Of Lead
Apr 23, 2025
-
Word Problems For Area Of A Triangle
Apr 23, 2025
Related Post
Thank you for visiting our website which covers about What Do Atoms Actually Look Like . We hope the information provided has been useful to you. Feel free to contact us if you have any questions or need further assistance. See you next time and don't miss to bookmark.