What Chemical Is Inside A Battery
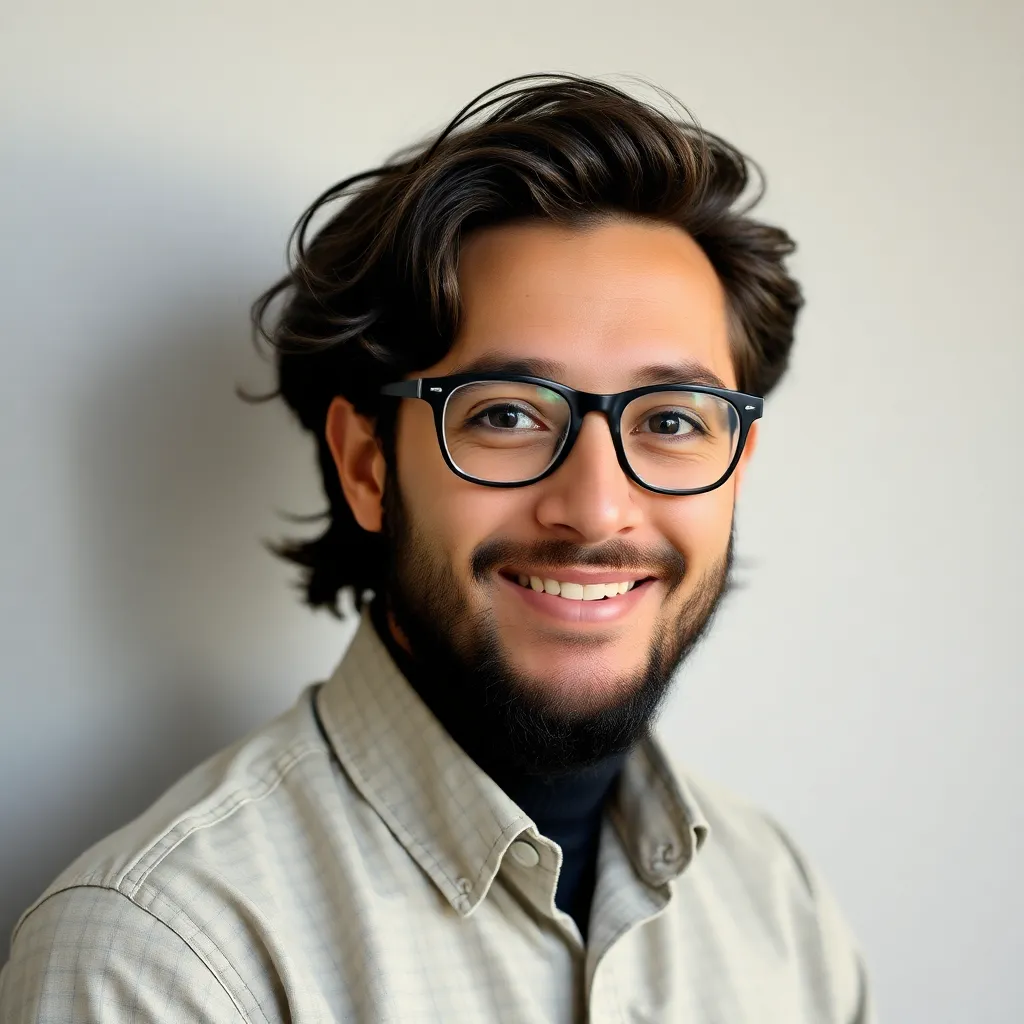
Juapaving
May 09, 2025 · 6 min read
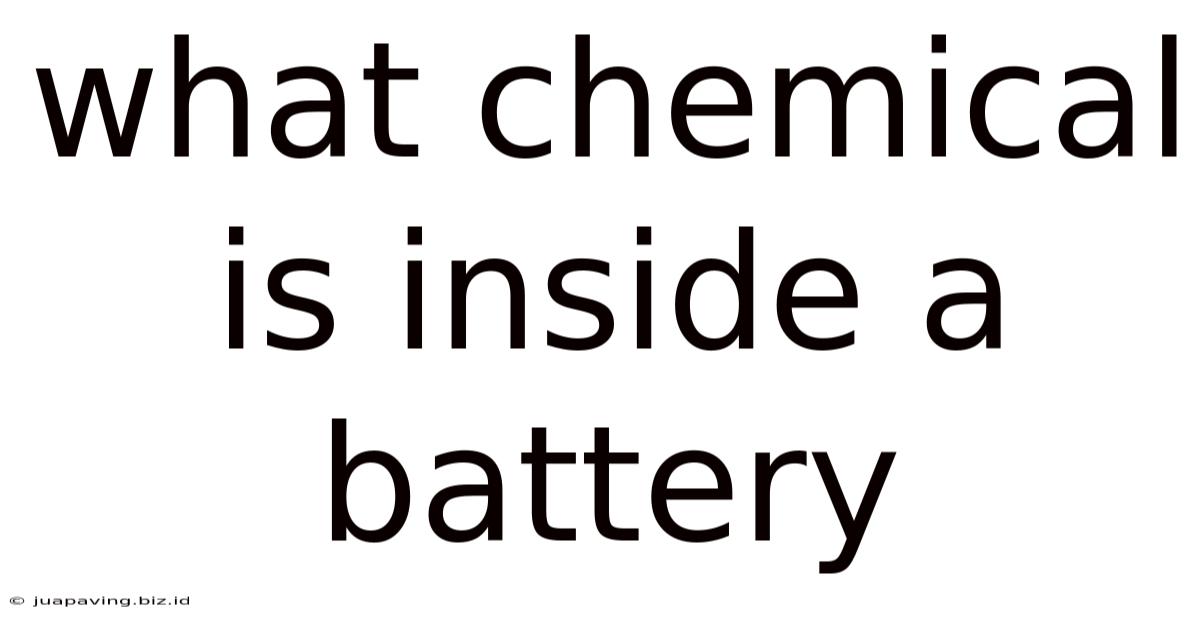
Table of Contents
What Chemical is Inside a Battery? A Deep Dive into Battery Chemistry
Batteries are ubiquitous in modern life, powering everything from smartphones and laptops to electric vehicles and grid-scale energy storage. But what exactly is inside these powerhouses? The answer is surprisingly complex, varying greatly depending on the type of battery. This article will delve into the chemical compositions of various battery types, exploring their strengths, weaknesses, and environmental implications.
Understanding Battery Basics: Electrolytes, Electrodes, and Reactions
Before exploring specific battery chemistries, let's establish some fundamental concepts. All batteries, regardless of type, share several key components:
1. Electrodes: The Powerhouses
Batteries have two electrodes: an anode (negative electrode) and a cathode (positive electrode). These electrodes are made of materials that readily participate in electrochemical reactions, facilitating the flow of electrons. The choice of electrode materials significantly influences the battery's voltage, energy density, and overall performance.
2. Electrolyte: The Conductor
The electrolyte is a conductive medium that allows ions (charged atoms) to move between the anode and cathode. This ion movement is crucial for completing the electrical circuit and enabling the battery to discharge (provide power) and charge (store energy). Electrolytes can be liquid, solid, or gel-like, each with its own properties and applications.
3. Separators: Maintaining Order
A separator is a porous membrane placed between the anode and cathode. Its primary role is to prevent direct contact between the electrodes, which would cause a short circuit and render the battery useless. The separator allows ion transport while physically separating the electrodes.
Common Battery Chemistries: A Detailed Look
Now, let's dive into the specific chemical compositions of some of the most prevalent battery types:
1. Alkaline Batteries (e.g., AA, AAA)
These are the most common primary (single-use) batteries. They are relatively inexpensive and readily available but have a lower energy density compared to rechargeable batteries.
- Anode: Zinc (Zn) powder. Zinc undergoes oxidation, releasing electrons.
- Cathode: Manganese dioxide (MnO₂) mixed with carbon black. Manganese dioxide undergoes reduction, accepting electrons.
- Electrolyte: Potassium hydroxide (KOH) solution. This alkaline electrolyte facilitates the movement of potassium and hydroxide ions between electrodes.
Chemical Reaction (Simplified): Zn + 2MnO₂ + H₂O → ZnO + Mn₂O₃ + 2OH⁻
Strengths: Inexpensive, readily available, good shelf life. Weaknesses: Low energy density, not rechargeable, relatively poor performance in low temperatures.
2. Lead-Acid Batteries (e.g., Car Batteries)
Lead-acid batteries are a mature technology, known for their high current output and durability. They are commonly used in vehicles and backup power systems.
- Anode: Lead (Pb) plates. Lead undergoes oxidation.
- Cathode: Lead dioxide (PbO₂) plates. Lead dioxide undergoes reduction.
- Electrolyte: Sulfuric acid (H₂SO₄) solution. Sulfuric acid participates in the electrochemical reaction and carries charge.
Chemical Reaction (Simplified): Pb + PbO₂ + 2H₂SO₄ ⇌ 2PbSO₄ + 2H₂O
Strengths: High current output, low cost, robust, relatively long lifespan. Weaknesses: Heavy, low energy density, requires regular maintenance, toxic materials.
3. Nickel-Cadmium (NiCd) Batteries
NiCd batteries were once popular, but their use has declined due to environmental concerns regarding cadmium's toxicity.
- Anode: Cadmium (Cd) metal.
- Cathode: Nickel oxyhydroxide (NiOOH).
- Electrolyte: Potassium hydroxide (KOH) solution.
Chemical Reaction (Simplified): Cd + 2NiOOH + 2H₂O ⇌ Cd(OH)₂ + 2Ni(OH)₂
Strengths: High energy density, long cycle life (number of charge-discharge cycles). Weaknesses: Cadmium toxicity, "memory effect" (reduced capacity if not fully discharged before recharging), relatively expensive.
4. Nickel-Metal Hydride (NiMH) Batteries
NiMH batteries are a significant improvement over NiCd, offering higher energy density and lacking the memory effect.
- Anode: A hydrogen-absorbing alloy (e.g., LaNi₅, AB₅ alloys). Hydrogen atoms are stored in the alloy's lattice.
- Cathode: Nickel oxyhydroxide (NiOOH).
- Electrolyte: Potassium hydroxide (KOH) solution.
Chemical Reaction (Simplified): MH + NiOOH ⇌ M + Ni(OH)₂
Strengths: Higher energy density than NiCd, no memory effect, environmentally friendly (compared to NiCd). Weaknesses: Lower voltage than NiCd, self-discharge rate is higher than NiCd.
5. Lithium-ion Batteries (Li-ion)
Li-ion batteries are currently the dominant battery technology for portable electronics and electric vehicles due to their high energy density, long cycle life, and relatively low self-discharge rate. However, there is a wide range of chemistries within the Li-ion family. Some common examples include:
- Lithium Cobalt Oxide (LCO): High energy density, but limited cycle life and safety concerns.
- Lithium Manganese Oxide (LMO): Lower energy density than LCO, but safer and more cost-effective.
- Lithium Nickel Manganese Cobalt Oxide (NMC): A balanced compromise between energy density, cost, and safety, widely used in EVs.
- Lithium Iron Phosphate (LFP): Excellent safety and long cycle life, but lower energy density.
General Components:
- Anode: Typically graphite, but silicon-based anodes are becoming more prevalent for improved energy density.
- Cathode: A transition metal oxide (e.g., LCO, LMO, NMC, LFP), containing lithium ions.
- Electrolyte: A lithium salt (e.g., LiPF₆) dissolved in an organic solvent.
Chemical Reaction (Simplified): LiC₆ (anode) + LiMO₂ (cathode) ⇌ 6C (anode) + Li₁₋ₓMO₂ (cathode) + xLi⁺ + xe⁻
Strengths: High energy density, long cycle life, low self-discharge rate, relatively lightweight. Weaknesses: Cost (depending on the cathode material), safety concerns (potential for thermal runaway), limited lifespan.
6. Solid-State Batteries
Solid-state batteries represent a promising future technology. They replace the liquid or gel electrolyte with a solid electrolyte, offering enhanced safety and potential for higher energy densities. However, they are currently in earlier stages of development and commercialization.
- Anode: Various materials, including lithium metal, graphite, or silicon.
- Cathode: Similar to Li-ion batteries (e.g., LCO, NMC, LFP).
- Electrolyte: A solid electrolyte, such as lithium sulfide (Li₂S), lithium phosphorus oxynitride (LiPON), or garnet-type ceramics.
Strengths: Enhanced safety (no flammable liquid electrolyte), potential for higher energy density, wider operating temperature range. Weaknesses: High manufacturing costs, limited cycle life in some designs, relatively low ionic conductivity (compared to liquid electrolytes) which can affect charging speed and performance.
Environmental Impact and Sustainability
The environmental impact of batteries is a significant concern, particularly concerning the extraction and processing of raw materials and the disposal of spent batteries. Different battery chemistries have varying environmental footprints.
- Heavy metals: Lead-acid and NiCd batteries contain toxic heavy metals, requiring careful recycling to prevent environmental contamination.
- Lithium: Lithium extraction can be environmentally demanding, with potential impacts on water resources and biodiversity.
- Manufacturing processes: The manufacturing of batteries can generate greenhouse gas emissions and consume significant energy.
Sustainable battery technology requires a holistic approach that considers the entire lifecycle, from raw material sourcing to end-of-life management. Recycling and reuse are crucial for minimizing the environmental footprint of batteries.
The Future of Battery Chemistry: Innovation and Advancements
Research and development in battery chemistry are constantly pushing the boundaries of energy storage. Scientists and engineers are exploring new materials and technologies to improve battery performance, safety, and sustainability. Some key areas of focus include:
- Solid-state electrolytes: As discussed earlier, solid-state electrolytes offer significant safety and performance advantages.
- New cathode materials: The search for higher energy density cathodes continues, with explorations into various metal oxides and sulfides.
- Improved anode materials: Silicon-based anodes are gaining traction, offering increased capacity compared to traditional graphite anodes.
- Sustainable materials: Research is focusing on using more abundant and less environmentally damaging materials in battery production.
- Advanced battery management systems: Sophisticated systems to monitor and control battery charging and discharging can improve battery lifespan and safety.
Conclusion: A Powerhouse of Chemistry
The chemical compositions inside batteries are intricate and varied, driving the performance and applications of these essential devices. Understanding the specific chemistries of different battery types is crucial for choosing the right battery for a given application, assessing its environmental impact, and driving innovation in this critical technology. As research continues, the future of battery chemistry promises even more efficient, sustainable, and powerful energy storage solutions.
Latest Posts
Latest Posts
-
What Are The Equivalent Fractions For 1 2
May 09, 2025
-
Do Both Plant And Animal Cells Have Endoplasmic Reticulum
May 09, 2025
-
How Many Milliliters Are In 1 75 Liters
May 09, 2025
-
What Is The Biggest Element On The Periodic Table
May 09, 2025
-
Image Formation In A Plane Mirror
May 09, 2025
Related Post
Thank you for visiting our website which covers about What Chemical Is Inside A Battery . We hope the information provided has been useful to you. Feel free to contact us if you have any questions or need further assistance. See you next time and don't miss to bookmark.