What Are Cells In A Circuit
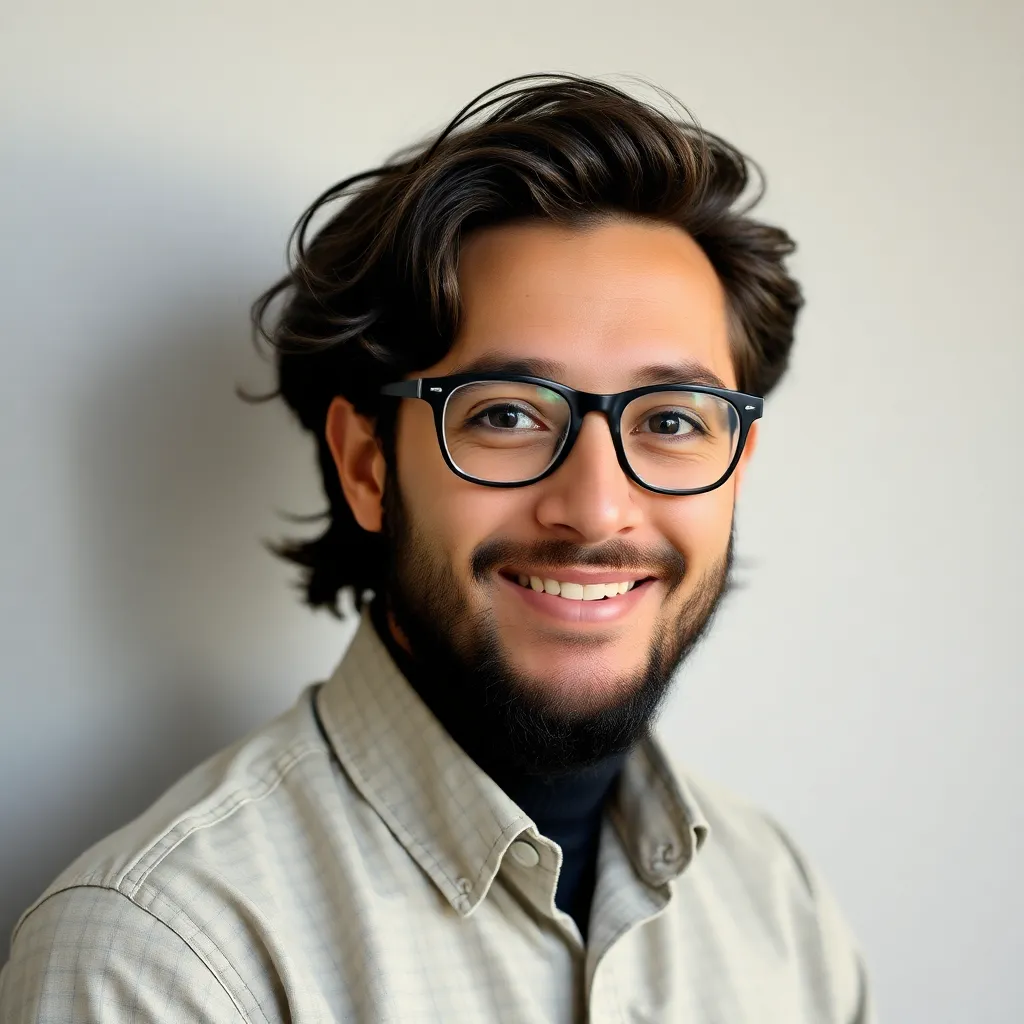
Juapaving
May 14, 2025 · 7 min read
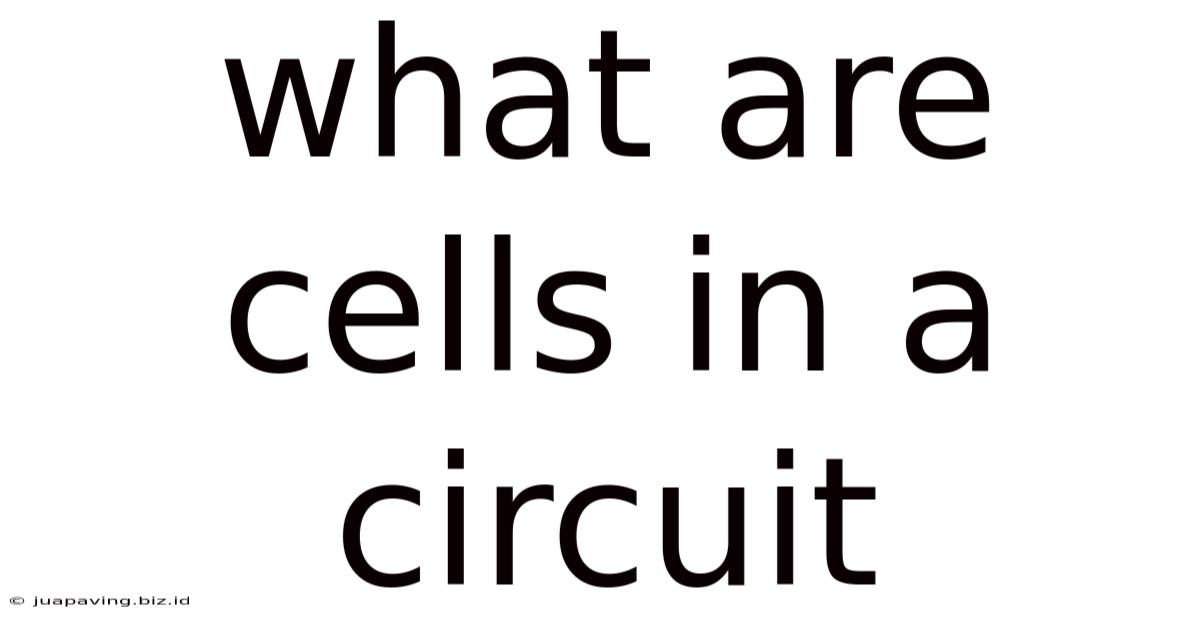
Table of Contents
What Are Cells in a Circuit? A Deep Dive into the Powerhouse of Electronics
Understanding the fundamental components of electrical circuits is crucial for anyone delving into electronics, whether you're a seasoned engineer or a curious beginner. At the heart of many circuits lies the humble cell, the basic unit of electrical energy. This comprehensive guide will delve into the intricacies of cells, exploring their types, functions, and their vital role in powering our electronic world.
What is a Cell? The Basic Building Block of Electricity
In the simplest terms, a cell is a device that converts chemical energy into electrical energy. It does this through a chemical reaction within its structure, creating a potential difference—a voltage—between two terminals: the positive (+) terminal and the negative (−) terminal. This voltage difference drives the flow of electrons, forming an electric current when connected to a complete circuit. Think of it as a tiny, self-contained battery.
Key Characteristics of a Cell:
-
Voltage (Electromotive Force - EMF): This is the potential difference measured in volts (V) between the cell's terminals. It represents the "push" the cell provides to the electrons, determining the current's strength. A higher voltage indicates a stronger push.
-
Current: This is the flow of electrons, measured in amperes (A) or amps. It's the rate at which electric charge flows through the circuit. The current depends on both the cell's voltage and the resistance of the circuit.
-
Internal Resistance: Even ideal cells have a small amount of internal resistance, which opposes the flow of current within the cell itself. This resistance consumes some of the energy generated, reducing the available voltage at the terminals.
-
Capacity: This refers to the amount of electrical energy a cell can store and deliver before it's depleted. It's usually measured in milliampere-hours (mAh) or ampere-hours (Ah). A higher capacity means the cell can power a device for a longer time.
-
Terminal Voltage: This is the actual voltage available at the terminals of the cell when current is flowing. It is always slightly lower than the EMF due to the internal resistance. The difference between the EMF and terminal voltage increases with increasing current draw.
Types of Cells: A Spectrum of Power Sources
Cells come in various shapes, sizes, and chemistries, each with unique properties and applications. The choice of cell depends on the specific requirements of the circuit, such as voltage, current, capacity, and cost.
1. Primary Cells (Non-Rechargeable):
These cells are designed for single use. Once their chemical energy is depleted, they cannot be recharged. They are commonly used in devices where replacing the cell is more practical than recharging it.
-
Zinc-Carbon Cells: These are the most common and inexpensive primary cells. They have a relatively low voltage (typically 1.5V) and limited capacity.
-
Alkaline Cells: These offer a higher energy density and longer lifespan compared to zinc-carbon cells, making them a popular choice for many everyday devices. They also provide a more stable voltage output.
-
Lithium Primary Cells: These cells boast significantly higher energy density than alkaline or zinc-carbon cells, making them ideal for applications requiring long-lasting power in compact devices. Different lithium chemistries exist, each with varying voltage and performance characteristics.
2. Secondary Cells (Rechargeable):
These cells can be recharged multiple times by reversing the chemical reaction that produces electricity. They are essential for portable electronics and many other applications.
-
Lead-Acid Cells: These are commonly used in car batteries and other heavy-duty applications. They offer high capacity but are relatively bulky and have a lower energy density compared to other rechargeable options.
-
Nickel-Cadmium (NiCd) Cells: These were popular for a time but are less common now due to environmental concerns related to cadmium. They have a "memory effect," meaning their capacity can decrease if not fully discharged before recharging.
-
Nickel-Metal Hydride (NiMH) Cells: These offer a higher energy density than NiCd cells and lack the memory effect. They are a popular choice for many rechargeable applications.
-
Lithium-ion (Li-ion) Cells: These cells dominate the rechargeable battery market due to their high energy density, long lifespan, and relatively low self-discharge rate. They are used extensively in smartphones, laptops, electric vehicles, and many other devices. Different lithium-ion chemistries exist, such as Lithium Cobalt Oxide (LCO), Lithium Manganese Oxide (LMO), Lithium Iron Phosphate (LFP), and Lithium Nickel Manganese Cobalt Oxide (NMC). Each chemistry offers a unique balance of energy density, lifespan, safety, and cost.
Cells in Series and Parallel: Combining Power Sources
Often, a single cell doesn't provide the required voltage or capacity for a particular application. In such cases, cells can be connected in series or parallel to achieve the desired characteristics.
1. Series Connection:
When cells are connected in series, their voltages add up. This increases the overall voltage of the circuit but keeps the current capacity the same as that of a single cell. For example, connecting two 1.5V cells in series results in a 3V source. This configuration is useful when a higher voltage is needed.
2. Parallel Connection:
Connecting cells in parallel increases the overall current capacity of the circuit without changing the voltage. The voltage remains the same as that of a single cell, but the combined capacity is the sum of the individual cell capacities. This configuration is beneficial when a higher current is needed for a longer duration.
Cells and Circuit Components: An Interplay of Energy and Resistance
Cells are rarely used in isolation. They are integrated within circuits along with various components, such as resistors, capacitors, and transistors. These components interact with the energy provided by the cell to perform specific functions.
-
Resistors: Resistors control the flow of current in a circuit. They consume some of the energy from the cell, converting it into heat. The amount of current that flows is determined by Ohm's Law (V = IR), where V is voltage, I is current, and R is resistance.
-
Capacitors: Capacitors store electrical energy in an electric field. They can temporarily provide current even when the cell is disconnected, useful for smoothing out voltage fluctuations or temporarily powering a circuit.
-
Transistors: Transistors act as electronic switches or amplifiers. They control the flow of current in a circuit based on the input signal. They rely on the voltage provided by the cell to operate.
Common Applications of Cells: Powering Our Modern World
The versatility of cells makes them an integral part of countless devices and systems in our modern world.
-
Portable Electronics: Smartphones, laptops, tablets, and other portable devices heavily rely on rechargeable cells (typically Li-ion) for their power.
-
Remote Controls: Many remote controls use smaller primary cells like alkaline or zinc-carbon cells.
-
Automotive: Lead-acid batteries power the starting, lighting, and ignition (SLI) systems in vehicles.
-
Medical Devices: Implantable medical devices like pacemakers and insulin pumps rely on specialized cells for long-term power.
-
Power Tools: Cordless power tools such as drills and saws utilize rechargeable batteries, usually NiMH or Li-ion.
-
Toys and Games: Numerous toys and electronic games utilize a variety of cell types depending on their power requirements.
-
Flashlights and Torches: These often employ primary cells like alkaline cells, offering convenience and a long operational life.
-
Emergency Lighting: Backup power systems in emergency lights utilize cells to provide illumination during power outages.
Safety Precautions When Handling Cells: Avoiding Hazards
While cells are essential for modern technology, it's crucial to handle them safely. Improper handling can lead to hazards:
-
Short Circuits: Never directly connect the positive and negative terminals of a cell. This can cause excessive current flow, leading to overheating, fire, and even explosion.
-
Overcharging: Overcharging rechargeable cells can damage them and potentially create safety risks. Always use an appropriate charger and follow manufacturer's instructions.
-
Disposal: Proper disposal of used cells is important for environmental reasons. Dispose of them according to local regulations.
Conclusion: Cells – The Unsung Heroes of Electronics
Cells are the fundamental power sources driving the electronic devices and systems that permeate our daily lives. Understanding their characteristics, types, and proper handling is crucial for anyone working with electronics or simply wanting to comprehend the technology around them. From the simple 1.5V battery in a flashlight to the sophisticated lithium-ion batteries in electric cars, cells remain the unsung heroes of our increasingly electrified world. By understanding the nuances of cell technology, we can better appreciate the power and potential of this seemingly simple yet incredibly vital component.
Latest Posts
Latest Posts
-
Will Stainless Steel Stick To A Magnet
May 14, 2025
-
What Is The Difference Between Renewable And Nonrenewable
May 14, 2025
-
How Many Kilograms Is 200 Pounds
May 14, 2025
-
How Much Is 168 Cm In Ft
May 14, 2025
-
How Many Litres Is 7 Gallons
May 14, 2025
Related Post
Thank you for visiting our website which covers about What Are Cells In A Circuit . We hope the information provided has been useful to you. Feel free to contact us if you have any questions or need further assistance. See you next time and don't miss to bookmark.