The Plasma Membrane Of An Axon Is Called The
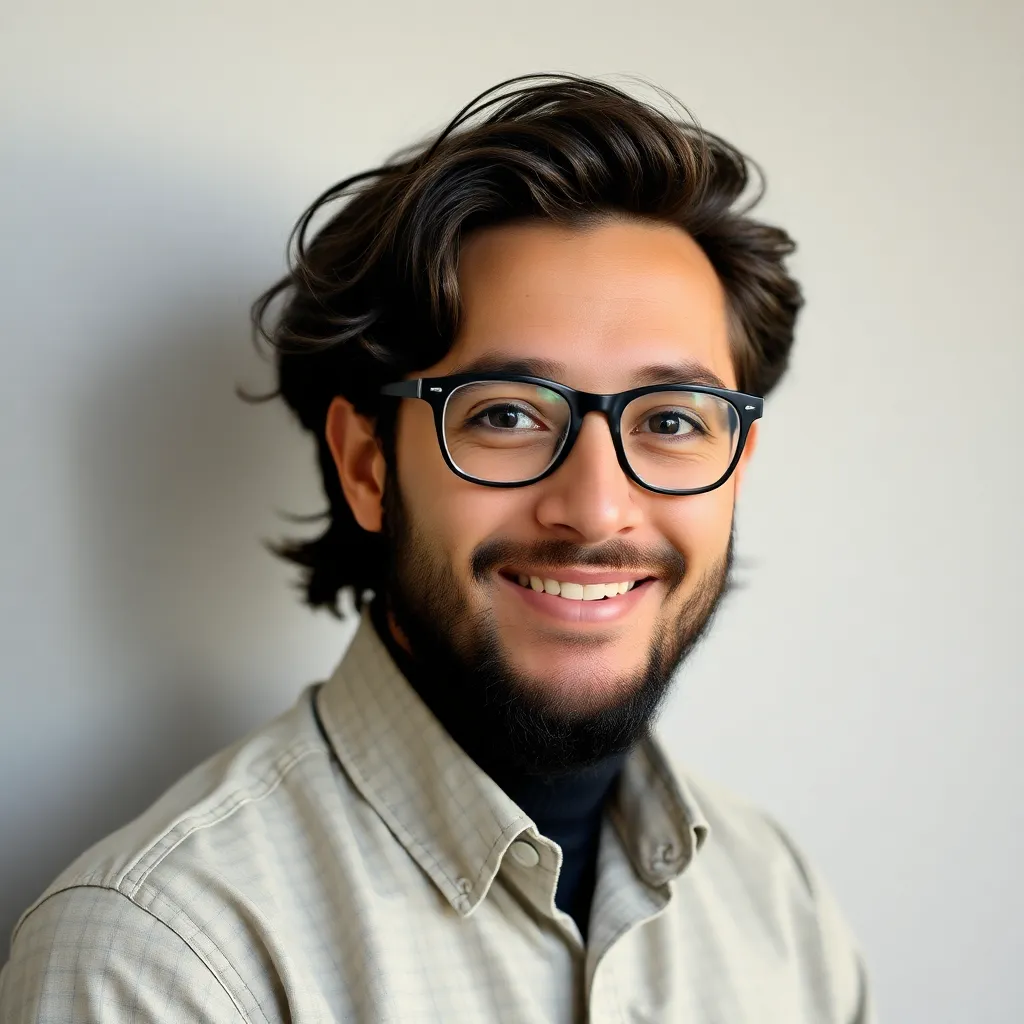
Juapaving
May 23, 2025 · 6 min read
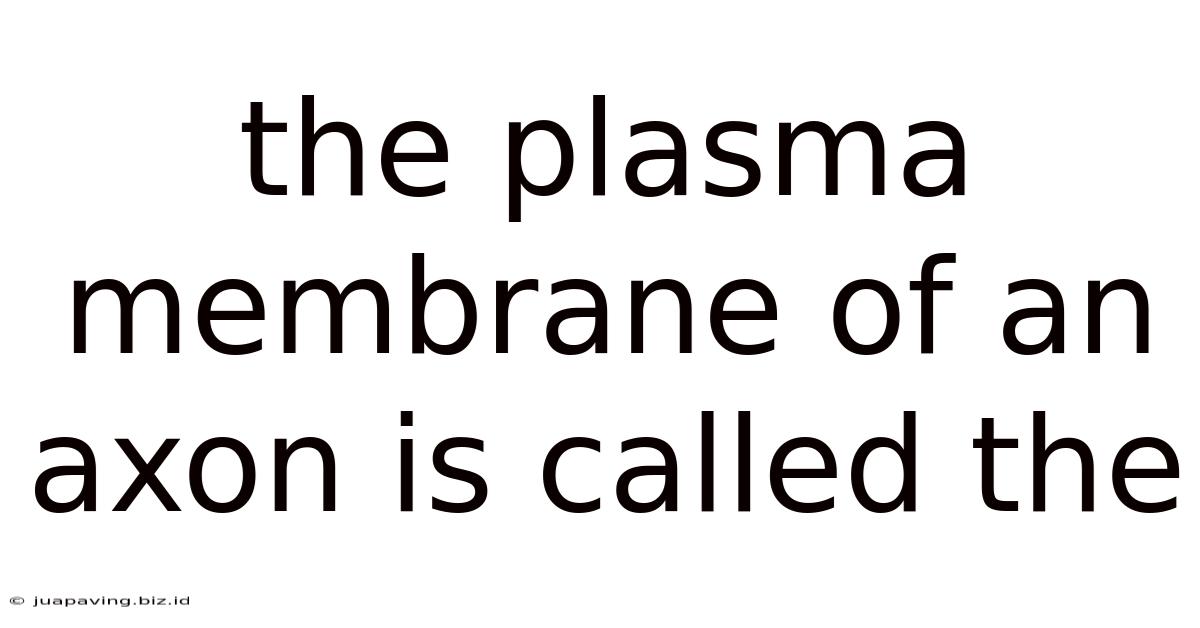
Table of Contents
The Plasma Membrane of an Axon is Called the Axolemma: A Deep Dive into Neuronal Structure and Function
The nervous system, a marvel of biological engineering, relies on the precise transmission of electrical and chemical signals to orchestrate bodily functions. At the heart of this intricate communication network lies the neuron, a specialized cell responsible for receiving, processing, and transmitting information. Understanding the neuron's structure is paramount to comprehending its function, and a key component of this structure is the axon, a long, slender projection extending from the neuron's cell body. The plasma membrane surrounding this crucial axon is specifically termed the axolemma. This article will explore the axolemma in detail, examining its composition, function, and significance in neuronal signaling.
The Axolemma: More Than Just a Membrane
The axolemma isn't just a passive barrier; it's a dynamic, highly specialized structure crucial for neuronal communication. It's a lipid bilayer, similar to the plasma membrane of other cells, but with a unique composition tailored to its specific role in action potential propagation. This composition includes:
1. Phospholipids: The Foundation
The axolemma's foundation, like all biological membranes, is a phospholipid bilayer. This bilayer consists of two layers of phospholipid molecules, each with a hydrophilic (water-loving) head and two hydrophobic (water-fearing) tails. This arrangement creates a selectively permeable barrier, controlling the movement of ions and molecules crucial for nerve impulse transmission. The specific types and ratios of phospholipids in the axolemma are meticulously regulated and contribute to its fluidity and stability.
2. Cholesterol: Maintaining Fluidity and Stability
Cholesterol molecules are interspersed within the phospholipid bilayer. These molecules play a vital role in maintaining membrane fluidity and stability. At lower temperatures, cholesterol prevents the phospholipids from packing too tightly, ensuring membrane fluidity. Conversely, at higher temperatures, cholesterol restricts excessive movement, preventing membrane disruption. This delicate balance is crucial for optimal axonal function.
3. Proteins: The Functional Workhorses
Proteins embedded within the axolemma are responsible for a wide range of crucial functions. These proteins can be broadly classified into:
a) Ion Channels: These are transmembrane proteins that form pores allowing specific ions (such as sodium (Na+), potassium (K+), calcium (Ca2+), and chloride (Cl−)) to pass across the membrane. The axolemma is particularly rich in voltage-gated ion channels, which open and close in response to changes in the membrane potential. These channels are pivotal for the generation and propagation of action potentials – the electrical signals that neurons use to communicate. Different types of voltage-gated channels exhibit varying activation thresholds and kinetics, contributing to the complexity and precision of neuronal signaling. Specific examples include:
- Voltage-gated sodium channels: Responsible for the rapid depolarization phase of the action potential.
- Voltage-gated potassium channels: Responsible for the repolarization phase of the action potential.
- Voltage-gated calcium channels: Play crucial roles in neurotransmitter release at the axon terminal.
b) Ion Pumps: Unlike ion channels, ion pumps actively transport ions across the membrane against their concentration gradients. This transport requires energy, typically in the form of ATP. The most prominent example in the axolemma is the sodium-potassium pump (Na+/K+ ATPase). This pump maintains the resting membrane potential by pumping three sodium ions out of the axon for every two potassium ions pumped in. This creates an electrochemical gradient crucial for action potential generation.
c) Receptors: The axolemma can also contain receptors that bind to neurotransmitters or other signaling molecules. While less prevalent on the axon compared to the dendrites and soma, these receptors can modulate axonal excitability and influence signal transmission.
d) Cell Adhesion Molecules (CAMs): CAMs are crucial for maintaining the structural integrity of the axon and its interactions with other cells in the nervous system. They facilitate connections between axons and glial cells, ensuring the proper myelination of axons in the peripheral and central nervous systems. Different types of CAMs contribute to the axolemma's structural organization and stability.
The Myelin Sheath and Nodes of Ranvier: Enhancing Axonal Conduction
Many axons, particularly those in the peripheral and central nervous systems, are wrapped in a myelin sheath. This insulating layer, formed by glial cells (oligodendrocytes in the CNS and Schwann cells in the PNS), dramatically increases the speed of action potential propagation. The myelin sheath is not continuous; it's interrupted at regular intervals by gaps called Nodes of Ranvier. These nodes are rich in voltage-gated ion channels, allowing for saltatory conduction – the rapid jumping of action potentials from one node to the next. The axolemma at the Nodes of Ranvier plays a critical role in this process.
Axolemma's Role in Action Potential Propagation
The axolemma's composition and structure directly contribute to its crucial role in action potential propagation:
-
Establishment of Resting Membrane Potential: The selective permeability of the axolemma, coupled with the activity of the Na+/K+ pump, establishes the resting membrane potential – a negative voltage across the membrane. This potential is essential for the initiation of an action potential.
-
Depolarization: Upon receiving a sufficient stimulus, voltage-gated sodium channels in the axolemma open, allowing a rapid influx of sodium ions. This influx causes a dramatic depolarization – a reversal of the membrane potential from negative to positive.
-
Repolarization: Following depolarization, voltage-gated potassium channels open, allowing potassium ions to flow out of the axon. This efflux of potassium ions restores the negative membrane potential, repolarizing the membrane.
-
Hyperpolarization: Briefly, the membrane potential may become more negative than the resting potential (hyperpolarization) before returning to its resting state. This is partly due to the continued efflux of potassium ions and the inactivation of sodium channels.
-
Saltatory Conduction (in myelinated axons): In myelinated axons, the action potential jumps between Nodes of Ranvier, significantly increasing the speed of conduction. The axolemma at these nodes is crucial for this rapid propagation.
Axolemma and Neuronal Diseases
Disruptions in the axolemma's structure or function can lead to a range of neurological disorders. These disruptions can result from:
- Genetic mutations: Affecting the structure or function of ion channels, pumps, or other axolemma proteins. Examples include various channelopathies that cause epilepsy, cardiac arrhythmias, and other neurological problems.
- Autoimmune diseases: Where the immune system attacks components of the axolemma, leading to demyelination and impaired neuronal function. Multiple sclerosis is a prime example of such a disease.
- Trauma or injury: Physical damage to axons can disrupt the axolemma's integrity, leading to neuronal dysfunction.
- Neurotoxins: Certain toxins can target components of the axolemma, disrupting its function and causing neurological deficits.
Conclusion: The Axolemma – A Crucial Player in Neuronal Function
The axolemma, the plasma membrane of the axon, is far more than just a passive barrier. Its unique composition and structure are meticulously tailored to support its pivotal role in action potential propagation. The intricate interplay of ion channels, pumps, receptors, and structural proteins within the axolemma ensures the precise and efficient transmission of electrical signals, the cornerstone of neuronal communication. Understanding the axolemma's structure and function is crucial not only for comprehending the basic mechanisms of neuronal signaling but also for developing effective treatments for a range of neurological disorders affecting this vital component of the nervous system. Further research into the complexities of the axolemma promises to unlock even more insights into the workings of the brain and nervous system, paving the way for advancements in neuroscience and medicine.
Latest Posts
Latest Posts
-
This Year You Decide To Focus Your Efforts
May 23, 2025
-
Chemical Equilibrium And Le Chateliers Principle Lab
May 23, 2025
-
Data Erasure Software Uses Standards That Are Called
May 23, 2025
-
Spinal Cord And Spinal Nerves Quiz
May 23, 2025
-
Fill In The Missing Column Of The Following Truth Table
May 23, 2025
Related Post
Thank you for visiting our website which covers about The Plasma Membrane Of An Axon Is Called The . We hope the information provided has been useful to you. Feel free to contact us if you have any questions or need further assistance. See you next time and don't miss to bookmark.