The Genetic Makeup Of An Organism
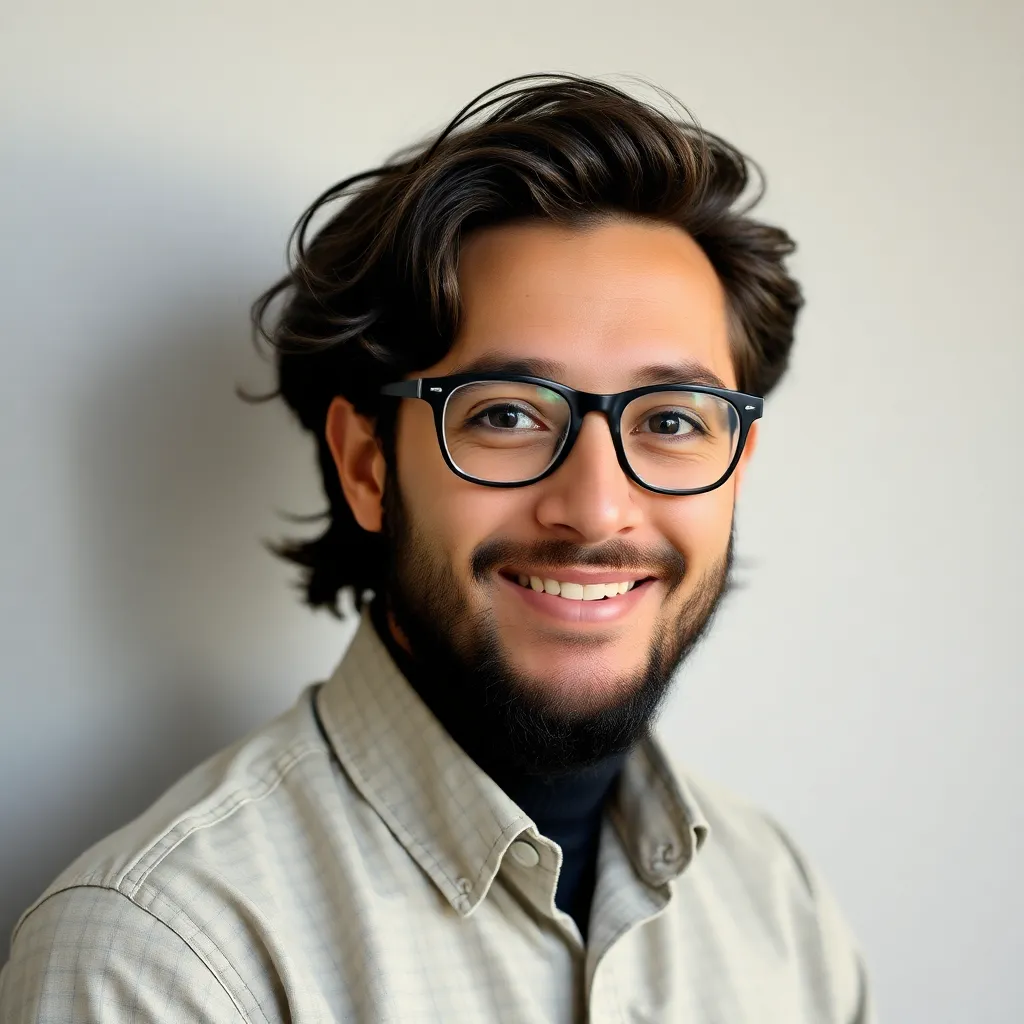
Juapaving
Apr 12, 2025 · 6 min read

Table of Contents
Decoding the Blueprint of Life: A Deep Dive into the Genetic Makeup of an Organism
The genetic makeup of an organism, often referred to as its genome, is the complete set of its genetic instructions. This intricate blueprint dictates every aspect of an organism's life, from its physical characteristics (phenotype) to its susceptibility to diseases. Understanding the genome is crucial to comprehending the complexities of life itself, unlocking the secrets of evolution, and developing revolutionary advancements in medicine and biotechnology. This article delves into the fascinating world of genetics, exploring the structure, function, and implications of an organism's genetic makeup.
The Fundamental Building Blocks: DNA and Genes
At the heart of every organism's genetic makeup lies deoxyribonucleic acid (DNA), a remarkable molecule shaped like a twisted ladder – the famous double helix. This double helix is composed of two strands of nucleotides, each consisting of a sugar molecule (deoxyribose), a phosphate group, and one of four nitrogenous bases: adenine (A), guanine (G), cytosine (C), and thymine (T). The order, or sequence, of these bases along the DNA strand is the fundamental code of life.
Within the vast DNA sequence, specific segments called genes encode the instructions for building and maintaining an organism. Each gene is a unit of heredity, containing the information necessary to synthesize a specific protein or functional RNA molecule. These proteins and RNA molecules perform a wide variety of functions within the cell, influencing everything from metabolism and cell signaling to development and reproduction.
The Central Dogma of Molecular Biology
The flow of genetic information follows the central dogma of molecular biology: DNA → RNA → Protein. This process begins with transcription, where the DNA sequence of a gene is copied into a messenger RNA (mRNA) molecule. The mRNA then travels to the ribosomes, where translation takes place. During translation, the mRNA sequence is "read" by the ribosome, and the corresponding amino acids are linked together to form a polypeptide chain, which folds into a functional protein.
Genome Organization and Variation
The organization of the genome varies greatly among organisms. In prokaryotes (bacteria and archaea), the genome is typically a single, circular chromosome located in the cytoplasm. Eukaryotes (plants, animals, fungi, and protists), on the other hand, have their DNA organized into multiple linear chromosomes located within the nucleus. These chromosomes are highly organized and compacted, ensuring that the vast amount of DNA can fit within the confines of the cell.
Genome size also varies tremendously across species. Some organisms have relatively small genomes, while others possess exceptionally large ones. This variation isn't necessarily correlated with the organism's complexity. For example, some plants have much larger genomes than humans. This difference can be attributed to variations in repetitive DNA sequences, which don't necessarily code for proteins but contribute to genome structure and regulation.
Furthermore, genomes are not static; they are constantly evolving. Genetic mutations, changes in the DNA sequence, are the raw material for evolution. These mutations can be caused by various factors, including errors during DNA replication, exposure to radiation, or chemical mutagens. Some mutations are harmful, while others are neutral or even beneficial, driving adaptation and speciation.
Beyond the Genes: Regulatory Elements and Non-Coding DNA
While genes are essential, they don't tell the whole story. The genome also includes numerous regulatory elements, sequences that control the expression of genes. These elements, such as promoters and enhancers, determine when, where, and to what extent genes are transcribed. Understanding the interplay between genes and their regulatory elements is crucial to deciphering the complex regulatory networks that govern cellular processes.
A significant portion of the genome, often referred to as non-coding DNA, doesn't directly code for proteins. However, this doesn't mean it's without function. Non-coding DNA plays a variety of roles, including:
- Gene regulation: Many non-coding sequences act as regulatory elements, controlling gene expression.
- Structural roles: Some non-coding DNA is involved in maintaining the structure and stability of chromosomes.
- RNA synthesis: Non-coding DNA can be transcribed into various types of functional RNA molecules, such as transfer RNA (tRNA) and ribosomal RNA (rRNA), essential for protein synthesis.
- Telomeres and centromeres: These specialized regions at the ends and centers of chromosomes are crucial for chromosome stability and replication.
The Human Genome: A Complex Tapestry
The human genome, comprising approximately 3 billion base pairs, is a testament to the complexity of life. It contains an estimated 20,000 to 25,000 protein-coding genes, representing only a small fraction of the total genome sequence. The vast majority of the human genome is composed of non-coding DNA, whose functions are still being actively researched.
The Human Genome Project, completed in 2003, provided a comprehensive map of the human genome, revolutionizing our understanding of human biology and disease. This project has laid the foundation for numerous advancements in medicine, including:
- Pharmacogenomics: Tailoring drug treatments to an individual's genetic makeup.
- Genetic testing: Identifying individuals at risk for certain diseases.
- Gene therapy: Developing treatments to correct genetic defects.
Understanding Genetic Variation and Disease
Genetic variation among individuals contributes to differences in their traits and susceptibility to diseases. Single nucleotide polymorphisms (SNPs), variations in a single nucleotide, are the most common type of genetic variation. These SNPs can affect gene expression and protein function, influencing an individual's phenotype and disease risk.
Many diseases, including cystic fibrosis, Huntington's disease, and sickle cell anemia, are caused by mutations in single genes. However, most complex diseases, such as heart disease, diabetes, and cancer, are influenced by multiple genes and environmental factors. Understanding the intricate interplay between genes and the environment is crucial for developing effective prevention and treatment strategies.
Epigenetics: Modifying Gene Expression Without Altering the DNA Sequence
Epigenetics is the study of heritable changes in gene expression that do not involve alterations to the underlying DNA sequence. These changes are often mediated by chemical modifications to DNA or histone proteins, which package and organize DNA within the chromosomes.
Epigenetic modifications can affect gene expression by altering the accessibility of DNA to transcription machinery. For example, DNA methylation, the addition of a methyl group to a cytosine base, can repress gene transcription. Histone modifications, such as acetylation and methylation, can also influence gene expression by affecting the structure of chromatin, the complex of DNA and proteins.
Epigenetic changes are influenced by various factors, including environmental exposures, diet, and lifestyle. These changes can be passed down through generations, potentially influencing the health and phenotypes of offspring. Epigenetics plays a critical role in development, aging, and disease, highlighting the dynamic interplay between genes and the environment.
The Future of Genomics: Unraveling the Mysteries of Life
The field of genomics is rapidly evolving, driven by technological advancements in DNA sequencing and bioinformatics. These advancements are paving the way for:
- Personalized medicine: Developing tailored treatments based on an individual's genetic profile.
- Precision agriculture: Optimizing crop yields and disease resistance through genetic engineering.
- Conservation biology: Understanding and protecting endangered species by analyzing their genetic diversity.
- Forensic science: Utilizing DNA analysis to solve crimes and identify individuals.
Understanding the genetic makeup of an organism is not merely an academic pursuit; it is crucial for addressing some of the most pressing challenges facing humanity. From developing cures for genetic diseases to understanding the intricacies of evolution, the insights gained from genomics are transforming our world in profound ways. The ongoing exploration of the genome promises further discoveries that will reshape our understanding of life and improve human health and well-being for generations to come.
Latest Posts
Latest Posts
-
What Goes Up And Downstairs Without Moving
Apr 18, 2025
-
How Many Centimeters Is 6 In
Apr 18, 2025
-
Who Is Considered The Father Of Sociology
Apr 18, 2025
-
Do Lysosomes Have A Double Membrane
Apr 18, 2025
-
Multiplying By A Multiple Of 10
Apr 18, 2025
Related Post
Thank you for visiting our website which covers about The Genetic Makeup Of An Organism . We hope the information provided has been useful to you. Feel free to contact us if you have any questions or need further assistance. See you next time and don't miss to bookmark.