The Complete Set Of Genes In An Organism
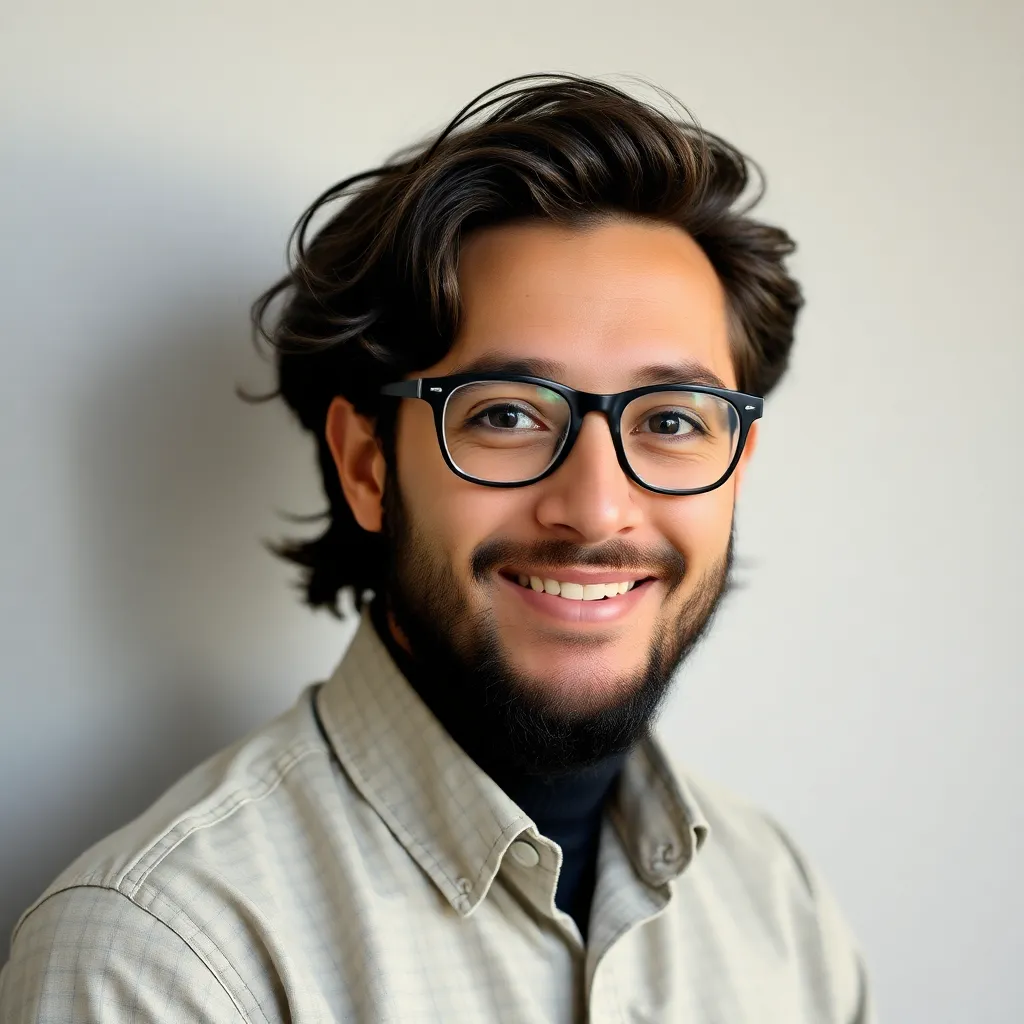
Juapaving
Apr 25, 2025 · 7 min read

Table of Contents
The Complete Set of Genes in an Organism: A Deep Dive into Genomes
The complete set of genes in an organism is known as its genome. This isn't just a simple list of genes; it's a complex and dynamic instruction manual, dictating everything from an organism's physical characteristics to its susceptibility to diseases. Understanding the genome is fundamental to advancing fields like medicine, agriculture, and evolutionary biology. This comprehensive article explores the intricacies of genomes, their structure, function, and the ever-evolving understanding of their role in shaping life as we know it.
What Exactly is a Genome?
A genome encompasses all the genetic material of an organism. This genetic material, primarily deoxyribonucleic acid (DNA), contains the instructions for building and maintaining the organism. While DNA is the most prevalent form of genetic material, some viruses use ribonucleic acid (RNA) as their genetic blueprint. Regardless of the type of genetic material, the genome holds the complete set of genes, along with non-coding DNA sequences that play crucial regulatory and structural roles.
The Size and Complexity of Genomes
Genome size varies tremendously across different species. A simple virus might have a genome consisting of only a few thousand base pairs (the building blocks of DNA), while the human genome boasts over 3 billion base pairs. This difference in size doesn't always correlate directly with organismal complexity. Some plants and amphibians have genomes significantly larger than the human genome. The concept of "junk DNA" – non-coding DNA sequences once thought to be functionless – is now understood to play vital roles in gene regulation and other cellular processes. The complexity of a genome is determined not just by size, but also by the number and arrangement of genes, regulatory elements, and repetitive sequences.
Exploring the Structure of Genomes: Genes and Beyond
The genome isn't a simple linear sequence of genes; its structure is intricate and hierarchical.
Genes: The Units of Heredity
Genes are the fundamental units of heredity. Each gene holds the instructions for building a specific protein or functional RNA molecule. These proteins and RNA molecules perform a vast array of functions within the cell, influencing the organism's traits and characteristics. Genes are comprised of coding sequences (exons) that are transcribed into RNA, and non-coding sequences (introns) that are spliced out during RNA processing. This process allows for alternative splicing, resulting in multiple protein isoforms from a single gene, further increasing the complexity of gene expression.
Non-Coding DNA: The Unsung Heroes
A significant portion of the genome doesn't directly code for proteins. This non-coding DNA includes:
- Regulatory sequences: These DNA segments control gene expression by influencing when, where, and to what extent genes are transcribed. They include promoters, enhancers, and silencers.
- Repetitive sequences: These are DNA segments that are repeated many times throughout the genome. Some repetitive sequences play roles in chromosome structure and stability, while others may be remnants of past genetic events.
- Introns: As mentioned earlier, these non-coding sequences within genes are removed during RNA processing.
- Pseudogenes: These are inactive gene copies that have accumulated mutations and lost their function. They can still provide valuable insights into gene evolution.
- Transposable elements: These are "jumping genes" that can move around the genome, sometimes disrupting gene function or creating new genetic variation.
Genome Function and Gene Expression
The genome's function is to provide the blueprint for building and maintaining an organism. This is achieved through a complex process called gene expression.
Transcription and Translation: From DNA to Protein
Gene expression begins with transcription, where the DNA sequence of a gene is copied into a messenger RNA (mRNA) molecule. This mRNA molecule then undergoes processing, including splicing to remove introns, before traveling to the ribosomes. At the ribosomes, the mRNA sequence is translated into a protein through a process involving transfer RNA (tRNA) molecules that carry amino acids, the building blocks of proteins.
Regulation of Gene Expression
Gene expression is tightly regulated to ensure that the right proteins are produced at the right time and in the right amount. This regulation occurs at multiple levels, including:
- Transcriptional regulation: This involves controlling the rate at which genes are transcribed into mRNA.
- Post-transcriptional regulation: This involves modifying the mRNA molecule after transcription, influencing its stability and translation efficiency.
- Translational regulation: This involves controlling the rate at which mRNA molecules are translated into proteins.
- Post-translational regulation: This involves modifying proteins after they have been synthesized, influencing their activity and stability.
Genome Evolution and Variation
Genomes are not static; they constantly evolve over time through various mechanisms:
Mutations: The Engine of Evolution
Mutations are changes in the DNA sequence. These can range from single base pair changes (point mutations) to large-scale chromosomal rearrangements. Mutations are the raw material of evolution, providing the variation upon which natural selection acts. Some mutations are neutral, having no significant effect on the organism, while others can be beneficial or harmful.
Recombination and Gene Flow
Recombination is the shuffling of genetic material during sexual reproduction. This process generates new combinations of alleles (different versions of a gene), increasing genetic diversity. Gene flow, the movement of genes between populations, also contributes to genetic diversity and adaptation.
Horizontal Gene Transfer
In prokaryotes (bacteria and archaea), horizontal gene transfer, the movement of genetic material between organisms other than through reproduction, is a significant mechanism of genome evolution. This can result in rapid adaptation to new environments or the acquisition of new functions.
Applications of Genome Knowledge
The understanding of genomes has revolutionized many fields:
Medicine: Diagnosis, Treatment, and Prevention
Genomics has transformed medical practice. Genome sequencing allows for the identification of genetic variations associated with diseases, leading to improved diagnostic tools, personalized medicine approaches tailored to an individual's genetic makeup, and the development of new therapeutic strategies. Genetic testing can help identify individuals at risk for certain diseases, allowing for proactive interventions and preventative measures.
Agriculture: Crop Improvement and Livestock Breeding
Genomics has revolutionized agriculture, enabling the development of crops with improved yield, disease resistance, and nutritional value. Genome sequencing allows for the identification of genes underlying desirable traits, enabling the development of genetically modified organisms (GMOs) and marker-assisted selection techniques to accelerate crop improvement. Similarly, genomics is used to enhance livestock breeding, leading to improved animal health, productivity, and meat quality.
Conservation Biology: Protecting Endangered Species
Genomic studies provide insights into the genetic diversity of endangered species, enabling the development of conservation strategies to protect genetic diversity and enhance population viability. Genome sequencing can reveal the genetic basis of adaptation to specific environments, helping in the design of effective conservation plans.
Forensic Science: Identifying Individuals and Solving Crimes
Genome sequencing plays a crucial role in forensic science, enabling the identification of individuals from DNA samples left at crime scenes. DNA profiling techniques are widely used in criminal investigations and paternity testing.
The Future of Genomics
The field of genomics is rapidly advancing, with new technologies continually improving our ability to sequence, analyze, and understand genomes. This includes:
- Next-Generation Sequencing (NGS): This technology allows for rapid and cost-effective sequencing of entire genomes, dramatically increasing our capacity to study genetic variation across populations and species.
- Genome Editing Technologies (CRISPR-Cas9): This revolutionary technology allows for precise modification of genomes, opening up new possibilities for treating genetic diseases and engineering organisms with desired traits.
- Metagenomics: This field studies the collective genomes of microbial communities, providing insights into the roles of microbes in various ecosystems and their impact on human health.
The ongoing research and technological advancements in genomics promise a future filled with even more profound understanding of life and its intricacies. From understanding the evolution of life on Earth to developing personalized medicine and sustainable agricultural practices, the applications of genome knowledge are vast and far-reaching. The continuous exploration of genomes will undoubtedly continue to reshape our understanding of the world around us and our place within it.
Latest Posts
Latest Posts
-
Modulus Of Elasticity Of Concrete Formula
Apr 25, 2025
-
What Is 35 Percent Of 75
Apr 25, 2025
-
What Are The Three Parts Of Dna
Apr 25, 2025
-
Least Common Multiple Of 25 And 35
Apr 25, 2025
-
Half Life Problems Worksheet And Answers
Apr 25, 2025
Related Post
Thank you for visiting our website which covers about The Complete Set Of Genes In An Organism . We hope the information provided has been useful to you. Feel free to contact us if you have any questions or need further assistance. See you next time and don't miss to bookmark.