Pyruvate Is Converted To Acetyl Coa In The
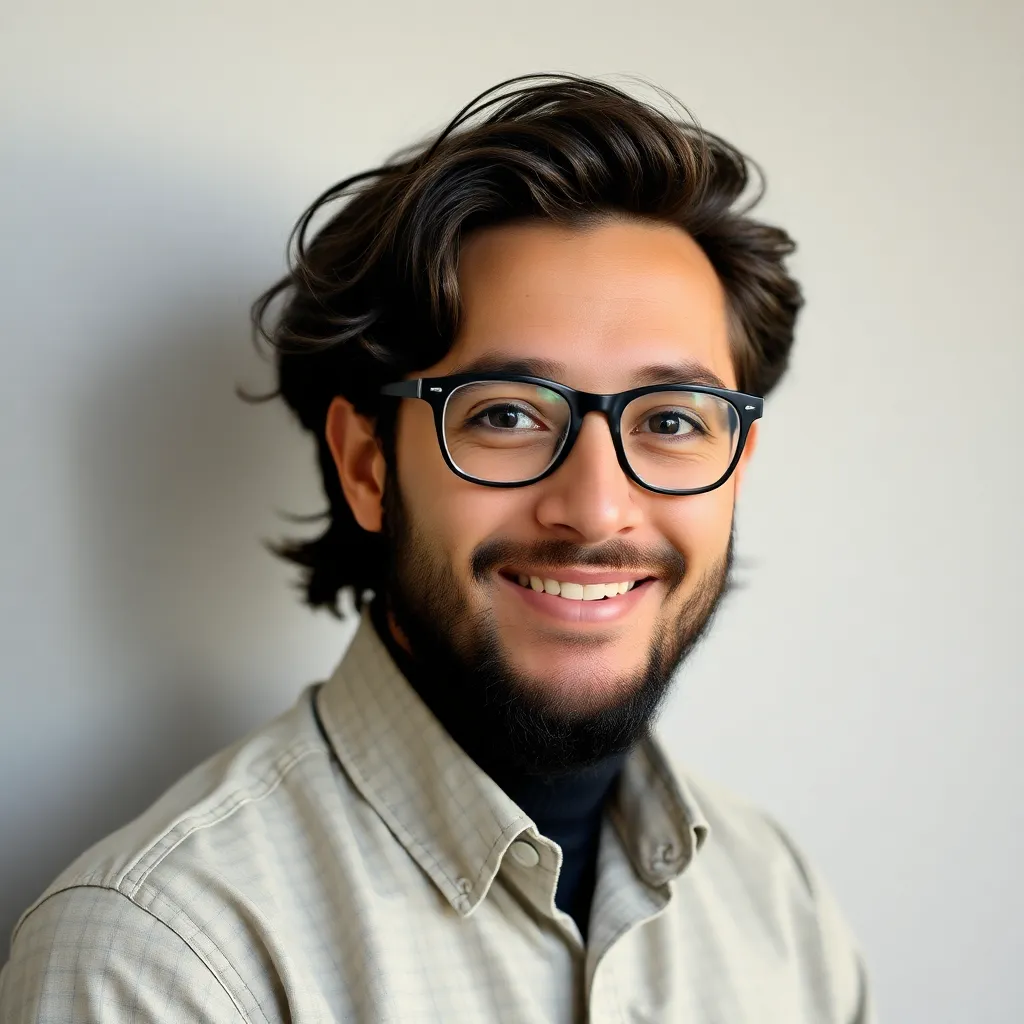
Juapaving
May 13, 2025 · 5 min read

Table of Contents
Pyruvate Is Converted to Acetyl-CoA in the Mitochondria: A Deep Dive into the Process
The conversion of pyruvate to acetyl-CoA is a pivotal step in cellular respiration, bridging glycolysis and the citric acid cycle (also known as the Krebs cycle or TCA cycle). This crucial reaction, occurring within the mitochondria, unlocks the vast energy potential stored within glucose and other metabolic fuels. Understanding this process is fundamental to grasping cellular energy production and its regulation.
The Location: The Mitochondrial Matrix
Before delving into the intricacies of the conversion, it's crucial to establish the location: the mitochondrial matrix. Mitochondria, often referred to as the "powerhouses" of the cell, are double-membraned organelles possessing their own DNA and ribosomes. The pyruvate-to-acetyl-CoA conversion takes place within the mitochondrial matrix, the innermost compartment of the mitochondrion. This specific location is vital because the enzymes involved in this reaction are resident within this space, and the products of this reaction feed directly into the citric acid cycle, also located within the matrix.
The Process: Pyruvate Dehydrogenase Complex (PDC) Action
The conversion of pyruvate to acetyl-CoA is not a single-step reaction but rather a multi-step process catalyzed by a large, multi-enzyme complex called the pyruvate dehydrogenase complex (PDC). This complex is a masterpiece of enzymatic coordination, bringing together several distinct enzymes and cofactors to efficiently convert pyruvate. The PDC is a remarkable example of metabolic efficiency, ensuring that the conversion proceeds smoothly and effectively.
The Five Key Enzymes and Their Roles:
The PDC consists of three core enzymes, each with specific roles:
-
Pyruvate dehydrogenase (E1): This enzyme catalyzes the first step, decarboxylating pyruvate to yield a two-carbon fragment, hydroxyethyl-TPP. Thiamine pyrophosphate (TPP), a derivative of vitamin B1, acts as a crucial cofactor in this step. A deficiency in vitamin B1 can impair PDC function.
-
Dihydrolipoyl transacetylase (E2): This enzyme transfers the two-carbon fragment from hydroxyethyl-TPP to lipoamide, a molecule containing a disulfide bond. This transfer generates acetyl-lipoamide. Lipoic acid is another vital cofactor in this reaction.
-
Dihydrolipoyl dehydrogenase (E3): This enzyme regenerates the oxidized form of lipoamide, completing the catalytic cycle and reducing NAD+ to NADH. FAD (flavin adenine dinucleotide) acts as a cofactor, receiving electrons from reduced lipoamide before transferring them to NAD+.
In addition to these three core enzymes, the PDC also includes two other crucial components:
-
Pyruvate dehydrogenase kinase: This enzyme regulates the activity of the PDC by phosphorylating and inactivating E1. This regulation is crucial for adjusting the rate of pyruvate metabolism based on cellular energy needs.
-
Pyruvate dehydrogenase phosphatase: This enzyme counteracts the action of the kinase by dephosphorylating and activating E1, thus restoring PDC activity.
The Coenzymes: Crucial Players in the Reaction
The PDC requires a complex interplay of five coenzymes for optimal function. These include:
- Thiamine pyrophosphate (TPP): Derived from vitamin B1, essential for the decarboxylation of pyruvate.
- Lipoic acid: A crucial cofactor for the transfer of the acetyl group.
- Coenzyme A (CoA-SH): Accepts the acetyl group to form acetyl-CoA, the final product.
- FAD (flavin adenine dinucleotide): A redox cofactor involved in electron transfer.
- NAD+ (nicotinamide adenine dinucleotide): Another redox cofactor that accepts electrons, generating NADH.
A deficiency in any of these coenzymes can significantly impair the PDC's activity, disrupting cellular energy metabolism.
The Regulation of Pyruvate Dehydrogenase Complex (PDC)
The activity of the PDC is tightly regulated to ensure that the conversion of pyruvate to acetyl-CoA aligns with the cell's energy demands. This regulation occurs at multiple levels:
-
Product inhibition: Acetyl-CoA and NADH, the products of the reaction, can inhibit the PDC's activity, preventing excessive acetyl-CoA production when cellular energy levels are high.
-
Substrate availability: The availability of pyruvate and CoA also influences the PDC's activity. High levels of pyruvate and CoA promote higher PDC activity.
-
Covalent modification: The phosphorylation of E1 by pyruvate dehydrogenase kinase inactivates the complex. Conversely, dephosphorylation by pyruvate dehydrogenase phosphatase reactivates the complex. This regulation is influenced by energy charge and the levels of several metabolites. High energy charge (high ATP and NADH levels) favors phosphorylation and inactivation of the PDC.
-
Allosteric regulation: Certain molecules can bind to allosteric sites on the PDC, influencing its activity. For example, ATP and acetyl-CoA inhibit the PDC, while ADP and CoA activate it.
This intricate regulatory system ensures that the conversion of pyruvate to acetyl-CoA is precisely tuned to the cell's metabolic needs.
The Significance of Acetyl-CoA
Acetyl-CoA is a central metabolic intermediate, playing a critical role in various metabolic pathways. Its primary function is as the initial substrate for the citric acid cycle, the main energy-generating pathway in aerobic respiration. Through the citric acid cycle, the acetyl group from acetyl-CoA is completely oxidized, generating high-energy molecules like NADH, FADH2, and GTP (guanosine triphosphate), which ultimately fuel ATP synthesis via oxidative phosphorylation.
The Fate of NADH
The NADH produced during the conversion of pyruvate to acetyl-CoA is another critical product. This molecule carries high-energy electrons that are subsequently transferred to the electron transport chain (ETC) located in the inner mitochondrial membrane. The ETC uses these electrons to generate a proton gradient across the membrane, which is harnessed by ATP synthase to produce ATP, the cell's primary energy currency.
Clinical Significance: PDC Deficiency
Defects in the pyruvate dehydrogenase complex (PDC) can lead to several serious metabolic disorders. These disorders, collectively known as pyruvate dehydrogenase complex deficiency (PDCD), result from mutations in the genes encoding the PDC enzymes or their associated cofactors. PDCD can manifest with a wide range of symptoms, from mild lactic acidosis to severe neurological problems, depending on the severity of the deficiency.
Conclusion: A Central Metabolic Hub
The conversion of pyruvate to acetyl-CoA is not merely a biochemical reaction; it is a critical metabolic crossroads. This process represents the transition from glycolysis to the citric acid cycle, playing a crucial role in energy production and metabolic regulation. Understanding the intricacies of the pyruvate dehydrogenase complex, its regulation, and its clinical significance is vital for appreciating the complexity and elegance of cellular metabolism. Further research continues to unveil deeper insights into this essential metabolic process, opening doors to potential therapeutic interventions for related metabolic disorders.
Latest Posts
Latest Posts
-
Electric Field From An Infinite Line Of Charge
May 13, 2025
-
What Is Red Phosphorus Phosphorous Bond Length
May 13, 2025
-
How Many People Can Fit In The Sun
May 13, 2025
-
What Are The Four Factors That Affect Climate
May 13, 2025
-
The Difference Between Heat And Temperature
May 13, 2025
Related Post
Thank you for visiting our website which covers about Pyruvate Is Converted To Acetyl Coa In The . We hope the information provided has been useful to you. Feel free to contact us if you have any questions or need further assistance. See you next time and don't miss to bookmark.