Predict The Final Product For The Following Synthetic Transformation
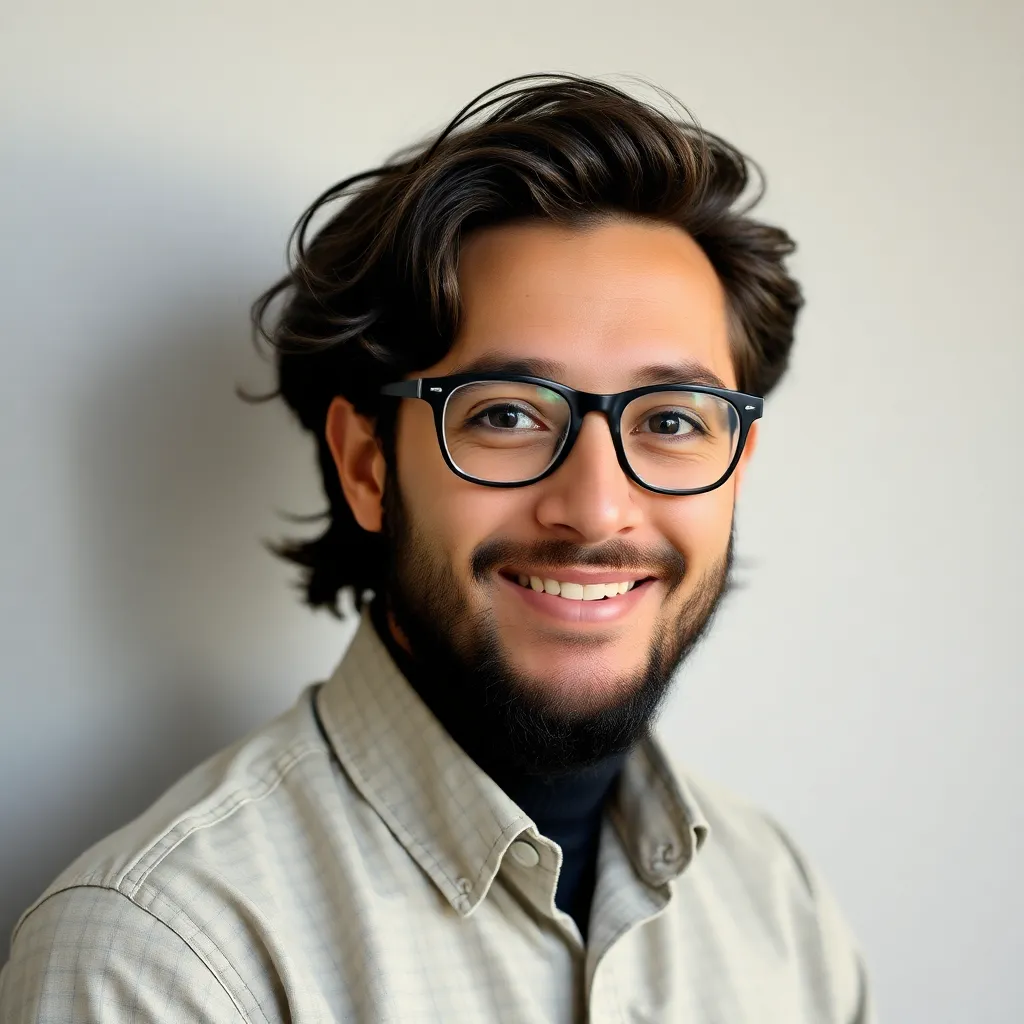
Juapaving
May 24, 2025 · 6 min read
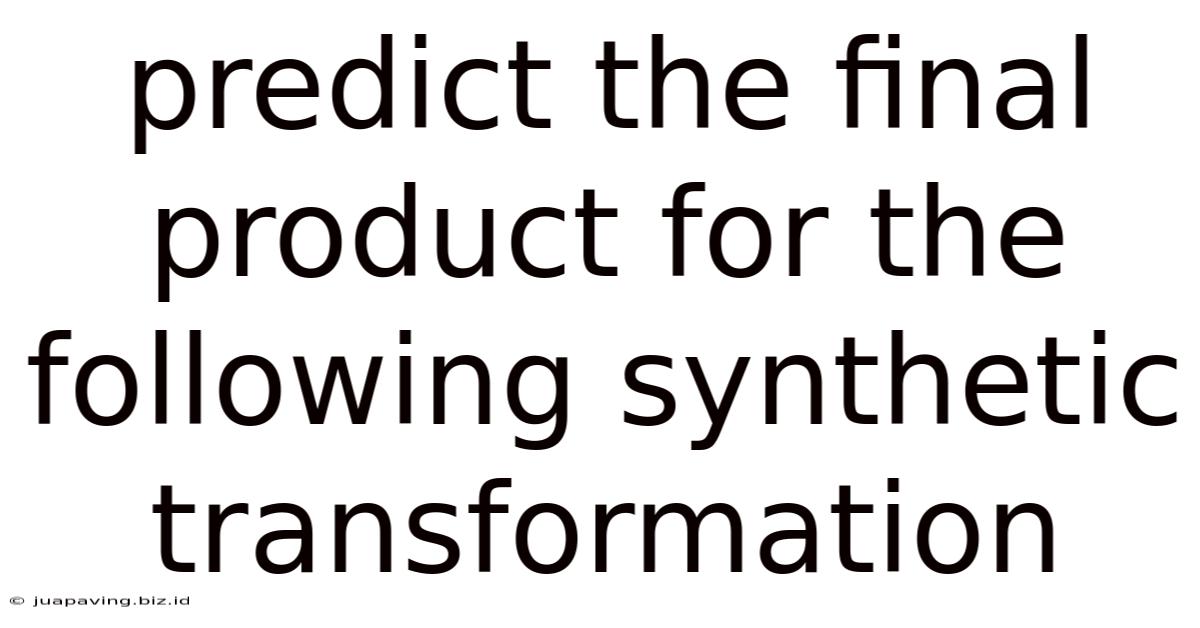
Table of Contents
Predicting the Final Product in Organic Synthesis: A Comprehensive Guide
Predicting the final product of a synthetic transformation is a cornerstone of organic chemistry. It requires a deep understanding of reaction mechanisms, functional group transformations, and the interplay of various reagents and conditions. This article will delve into the process of predicting outcomes for complex synthetic transformations, covering key principles and providing examples to solidify understanding. We’ll explore strategies for approaching such problems, from simple reactions to more intricate multi-step syntheses.
Understanding Reaction Mechanisms: The Foundation of Prediction
Before attempting to predict the final product of any synthetic transformation, a thorough understanding of the underlying reaction mechanism is paramount. This involves identifying the key steps, intermediates, and the driving forces behind the transformation. Different mechanisms, such as SN1, SN2, E1, E2, addition, elimination, and rearrangement reactions, lead to distinct product distributions.
1. Nucleophilic Substitution (SN1 & SN2): SN1 reactions proceed through a carbocation intermediate, making them sensitive to carbocation stability. Tertiary carbocations are more stable than secondary, which are more stable than primary. SN2 reactions proceed through a concerted mechanism, with inversion of stereochemistry at the reaction center. Steric hindrance around the reaction center significantly impacts the rate of SN2 reactions.
2. Elimination Reactions (E1 & E2): E1 reactions, like SN1, proceed through a carbocation intermediate, leading to potential for rearrangement. E2 reactions are concerted, requiring a specific anti-periplanar geometry between the leaving group and the proton being abstracted. The strength of the base and the steric environment around the substrate influence the selectivity of E2 reactions.
3. Addition Reactions: These reactions involve the addition of a reagent across a multiple bond (e.g., C=C, C≡C, C=O). The regioselectivity and stereoselectivity of addition reactions depend on factors such as the nature of the electrophile and the nucleophile, as well as the presence of any catalysts or directing groups. Markovnikov's rule and anti-Markovnikov addition are key concepts to consider.
4. Oxidation and Reduction Reactions: These reactions involve the change in oxidation state of atoms within a molecule. Predicting the outcome requires knowledge of the oxidizing or reducing agent’s strength and selectivity. Common oxidizing agents include potassium permanganate (KMnO4), chromic acid (H2CrO4), and Jones reagent (CrO3/H2SO4), while common reducing agents include lithium aluminum hydride (LiAlH4), sodium borohydride (NaBH4), and catalytic hydrogenation (H2/Pd).
5. Rearrangement Reactions: These reactions involve the reorganization of atoms within a molecule, often driven by the formation of a more stable structure. Common rearrangement reactions include Claisen rearrangement, Cope rearrangement, and Wagner-Meerwein rearrangement. Understanding the driving forces behind these rearrangements is crucial for accurate prediction.
Analyzing the Starting Material and Reagents: Key Considerations
Before diving into the mechanism, carefully analyze the starting material and the reagents used. Identify all functional groups present and their reactivity. Consider the steric factors influencing the reaction. The choice of reagents often dictates the reaction pathway.
1. Functional Group Reactivity: Different functional groups have characteristic reactivity. For example, alcohols can be oxidized to aldehydes or ketones, while alkenes can undergo addition, oxidation, or polymerization reactions. Understanding this reactivity is vital for anticipating the transformations.
2. Reagent Selectivity: Reagents often exhibit selectivity, favoring specific functional groups or reaction pathways. For example, DIBAL-H (diisobutylaluminum hydride) selectively reduces esters to aldehydes, while LiAlH4 reduces them to alcohols. Understanding this selectivity is crucial for controlling the reaction outcome.
3. Steric Effects: Steric hindrance can significantly influence reaction rates and selectivity. Bulky groups can hinder nucleophilic attack or proton abstraction, leading to different product distributions. Consider the steric environment around the reaction center when predicting the outcome.
4. Solvent Effects: The solvent used can influence the reaction pathway. Polar solvents favor polar reactions, while nonpolar solvents favor nonpolar reactions. The solvent can also affect the stability of intermediates, influencing the product distribution.
Multi-Step Syntheses: A Strategic Approach
Predicting the final product of a multi-step synthesis requires a systematic approach. Break down the synthesis into individual steps, predicting the product of each step before proceeding to the next. This iterative approach allows for a more accurate prediction of the overall outcome.
1. Retrosynthetic Analysis: This powerful technique involves working backward from the target molecule, identifying key precursors and the reactions needed to synthesize them. It provides a structured approach to designing complex syntheses.
2. Protecting Groups: In multi-step syntheses, protecting groups may be necessary to prevent unwanted reactions of certain functional groups. Predicting the need for protecting groups and choosing appropriate ones is crucial for achieving the desired outcome.
3. Reaction Optimization: Often, the reaction conditions need to be optimized to achieve the desired selectivity and yield. This may involve adjusting the temperature, solvent, or reagent stoichiometry. Predicting the effect of these changes requires a good understanding of the reaction mechanism and kinetics.
Illustrative Examples: Predicting Product Outcomes
Let's consider a few examples to illustrate the principles discussed above. Note: Without a specific synthetic transformation provided, I cannot offer specific predictions. Instead, I'll present generalized examples to showcase the process.
Example 1: SN2 Reaction
Consider the reaction of bromomethane (CH3Br) with sodium cyanide (NaCN) in DMF (dimethylformamide). This is a classic SN2 reaction. The cyanide ion (CN-) acts as a nucleophile, attacking the carbon atom bearing the bromine atom. The bromine atom leaves as a bromide ion. The product will be acetonitrile (CH3CN), with inversion of stereochemistry if the starting material were chiral.
Example 2: E2 Elimination
Consider the reaction of 2-bromobutane with a strong base like potassium tert-butoxide (t-BuOK). This will favor E2 elimination. The strong base will abstract a proton from a beta-carbon, leading to the formation of a double bond. The major product will be 2-butene (the more substituted alkene, due to Zaitsev's rule), with a possible minor product of 1-butene.
Example 3: Multi-step Synthesis
Imagine a synthesis involving the conversion of an alcohol to an alkene, followed by an epoxidation reaction. First, the alcohol would be converted to a good leaving group (e.g., via tosylation), followed by treatment with a strong base to effect elimination, forming the alkene. The alkene could then be epoxidized using a peroxyacid like mCPBA (meta-chloroperbenzoic acid), yielding the epoxide.
These are simplified examples; real-world synthetic transformations can be significantly more complex. However, the principles illustrated here provide a robust foundation for predicting the outcomes of a wide variety of reactions.
Conclusion: Mastering Prediction in Organic Synthesis
Predicting the final product of a synthetic transformation is a challenging but rewarding aspect of organic chemistry. By mastering reaction mechanisms, understanding the reactivity of functional groups and reagents, and employing systematic strategies like retrosynthetic analysis, chemists can accurately predict outcomes and design efficient synthetic routes. Continuous practice and a deep understanding of organic chemistry principles are essential for developing this crucial skill. Remember, detailed analysis of the specific reagents, conditions, and starting materials is always paramount to accurate prediction.
Latest Posts
Latest Posts
-
Where Does Hills Like White Elephants Take Place
May 24, 2025
-
Samsung Electronics Co Ltd Usb 2 19 1 0
May 24, 2025
-
How Many Cubic Feet Are In A 53 Foot Trailer
May 24, 2025
-
The Hunger Games Book Summary Chapter 1
May 24, 2025
-
Examples Of Optically Inactive Fisher Projection
May 24, 2025
Related Post
Thank you for visiting our website which covers about Predict The Final Product For The Following Synthetic Transformation . We hope the information provided has been useful to you. Feel free to contact us if you have any questions or need further assistance. See you next time and don't miss to bookmark.