Neuron Anatomy And Physiology Exercise 13
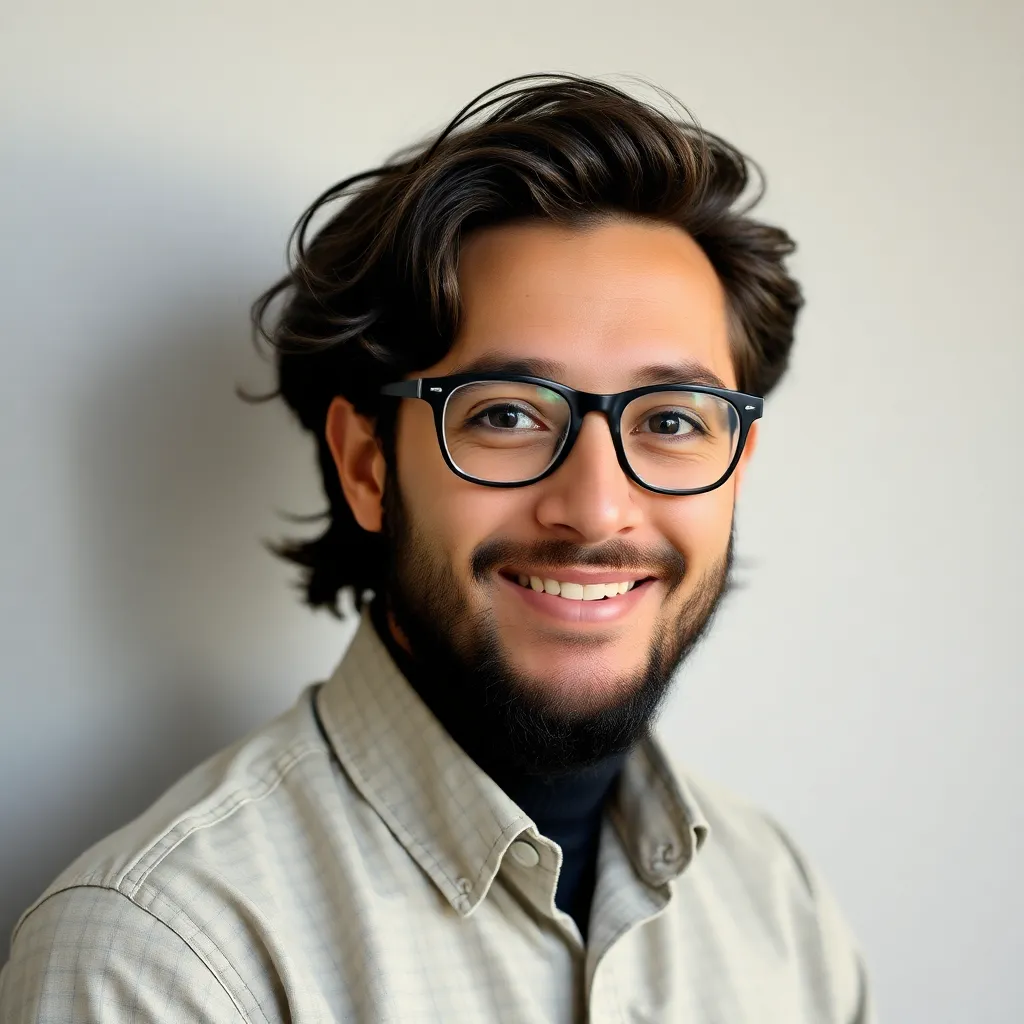
Juapaving
May 23, 2025 · 6 min read
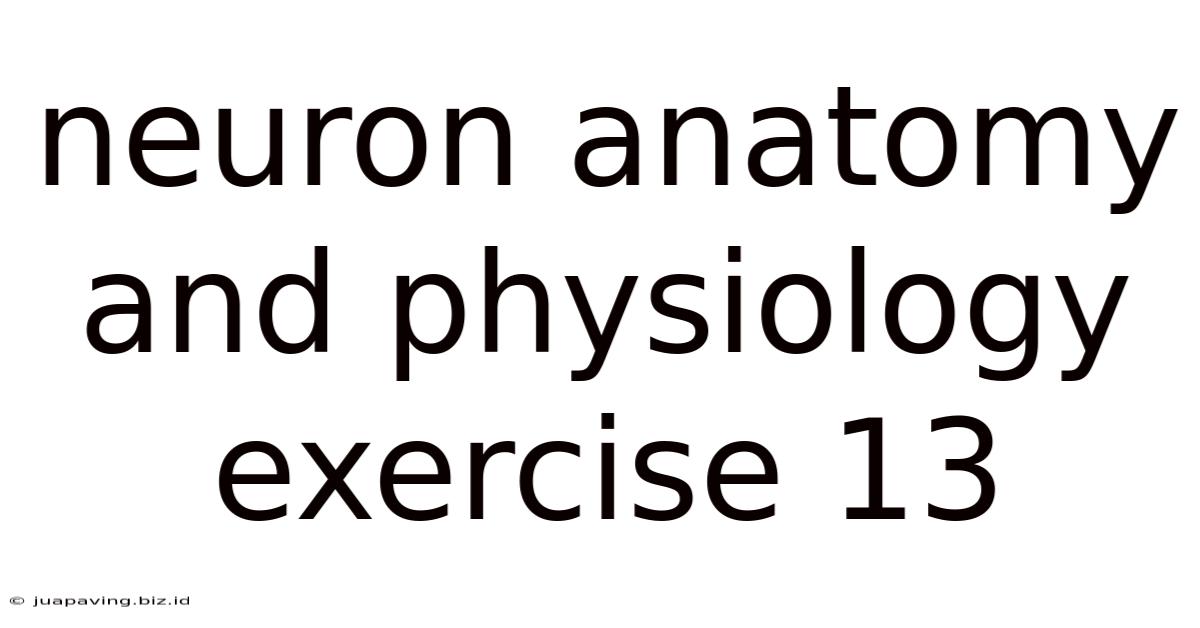
Table of Contents
Neuron Anatomy and Physiology Exercise 13: A Deep Dive into Neural Communication
This comprehensive guide delves into the intricacies of neuron anatomy and physiology, focusing on the key concepts relevant to Exercise 13 (assuming a specific exercise structure within a broader course). We will explore the structure of a neuron, its functional components, the mechanisms of neural transmission, and common scenarios encountered in studying neuronal activity. This detailed explanation aims to provide a robust understanding, aiding both learning and problem-solving in exercises related to this fascinating field.
Understanding the Neuron: The Building Block of the Nervous System
Before diving into the specifics of Exercise 13, let's establish a solid foundation in neuron structure and function. Neurons, the fundamental units of the nervous system, are specialized cells responsible for receiving, processing, and transmitting information throughout the body. This communication allows for coordinated actions, thoughts, and emotions.
Key Anatomical Components:
-
Dendrites: These branched extensions act as the neuron's primary receivers of signals. They receive neurotransmitters from other neurons across specialized junctions called synapses. The greater the dendritic branching, the more signals a neuron can receive and integrate.
-
Soma (Cell Body): The soma contains the neuron's nucleus and other essential organelles. It integrates the signals received from the dendrites. If the integrated signal surpasses a certain threshold, it initiates an action potential.
-
Axon: A long, slender projection extending from the soma. The axon transmits the action potential, a rapid electrical signal, away from the soma to other neurons or target cells. The axon's length varies greatly depending on the neuron's location and function.
-
Axon Hillock: This region connects the soma to the axon. It is the site where the decision to fire an action potential is made – the 'trigger zone'.
-
Myelin Sheath: Many axons are covered by a myelin sheath, a fatty insulating layer formed by glial cells (oligodendrocytes in the central nervous system and Schwann cells in the peripheral nervous system). The myelin sheath significantly speeds up the transmission of the action potential through saltatory conduction.
-
Nodes of Ranvier: Gaps in the myelin sheath where the axon membrane is exposed. These nodes are crucial for saltatory conduction, allowing the action potential to "jump" between nodes, accelerating transmission speed.
-
Axon Terminals (Synaptic Terminals/Boutons): These are the branches at the end of the axon where neurotransmitters are released into the synapse. The neurotransmitters then bind to receptors on the dendrites of the next neuron, continuing the signal transmission.
Neural Transmission: The Electrochemical Dance
Neural communication is a complex electrochemical process involving both electrical and chemical signals. Understanding this process is key to comprehending Exercise 13 and related concepts.
The Action Potential: An All-or-Nothing Event
The action potential is a rapid, self-propagating depolarization of the axon membrane. It follows an "all-or-nothing" principle: either an action potential occurs with full amplitude, or it doesn't occur at all. The intensity of the stimulus is not encoded in the amplitude of the action potential, but rather in the frequency of action potentials.
Stages of an Action Potential:
-
Resting Potential: The neuron maintains a negative resting membrane potential, typically around -70mV. This is maintained by the sodium-potassium pump, which actively transports ions across the membrane.
-
Depolarization: An incoming stimulus causes depolarization – a decrease in the membrane potential towards zero. If the depolarization reaches the threshold potential, an action potential is triggered.
-
Rising Phase: Voltage-gated sodium channels open rapidly, causing a massive influx of sodium ions into the axon. This further depolarizes the membrane, making it positive inside.
-
Falling Phase: Voltage-gated potassium channels open, allowing potassium ions to flow out of the axon. This repolarizes the membrane, returning it towards its resting potential.
-
Hyperpolarization: The potassium channels remain open slightly longer than necessary, causing a brief hyperpolarization (membrane potential more negative than resting potential).
-
Return to Resting Potential: The sodium-potassium pump restores the ion gradients, returning the membrane potential to its resting state.
Synaptic Transmission: The Chemical Handoff
Once the action potential reaches the axon terminals, it triggers the release of neurotransmitters. This process is known as synaptic transmission, a crucial chemical step in neural communication.
Steps of Synaptic Transmission:
-
Action Potential Arrival: The action potential reaches the axon terminal.
-
Calcium Influx: The depolarization opens voltage-gated calcium channels, allowing calcium ions to enter the axon terminal.
-
Neurotransmitter Release: The influx of calcium triggers the fusion of synaptic vesicles (containing neurotransmitters) with the presynaptic membrane.
-
Neurotransmitter Diffusion: Neurotransmitters are released into the synaptic cleft (the gap between the presynaptic and postsynaptic neurons).
-
Receptor Binding: Neurotransmitters diffuse across the cleft and bind to specific receptors on the postsynaptic membrane.
-
Postsynaptic Potential: Binding of neurotransmitters to receptors can either depolarize (excitatory postsynaptic potential, EPSP) or hyperpolarize (inhibitory postsynaptic potential, IPSP) the postsynaptic neuron.
-
Signal Termination: Neurotransmitters are removed from the synaptic cleft through reuptake, enzymatic degradation, or diffusion.
Exercise 13: Potential Scenarios and Problem-Solving
Without the exact content of Exercise 13, we can explore common scenarios encountered when studying neuron anatomy and physiology. These examples will help you understand the principles and apply them to the specific problems in your exercise.
Example Scenario 1: Analyzing Action Potential Graphs:
Exercise 13 might present graphs depicting action potentials. You would need to identify the phases (resting potential, depolarization, repolarization, hyperpolarization), understand the roles of ion channels, and possibly interpret changes resulting from pharmacological interventions or pathological conditions.
Example Scenario 2: Predicting the Effect of Neurotransmitter Release:
You might be presented with a scenario describing the release of a specific neurotransmitter (e.g., glutamate, GABA, acetylcholine) at a particular synapse. You need to predict the effect on the postsynaptic neuron (depolarization or hyperpolarization) based on the neurotransmitter's action (excitatory or inhibitory).
Example Scenario 3: Understanding Saltatory Conduction:
Exercise 13 could involve questions about the speed of conduction in myelinated versus unmyelinated axons. Understanding the mechanism of saltatory conduction and its dependence on the myelin sheath and Nodes of Ranvier is crucial.
Example Scenario 4: Interpreting the Effects of Ion Channel Blockers:
The exercise might involve scenarios where specific ion channels are blocked (e.g., sodium channels, potassium channels, calcium channels). You would need to predict the consequences of these blockages on the generation and propagation of action potentials and synaptic transmission.
Example Scenario 5: Analyzing Neurological Disorders:
Some exercises might focus on the link between neuron malfunction and neurological disorders. For example, understanding how multiple sclerosis (affecting myelin) or certain neurological diseases affecting ion channels manifest clinically.
Mastering Neuron Anatomy and Physiology: Tips and Strategies
To succeed in Exercise 13 and related coursework:
-
Active Recall: Regularly test your knowledge through active recall techniques, such as flashcards or practice questions. This strengthens memory and identifies knowledge gaps.
-
Visual Learning: Use diagrams and animations to visualize the structure of a neuron and the process of neural transmission.
-
Conceptual Understanding: Focus on understanding the underlying mechanisms rather than just memorizing facts. This allows for better problem-solving.
-
Practice Problems: Work through numerous practice problems to solidify your understanding and develop problem-solving skills.
-
Seek Clarification: Don't hesitate to ask your instructor or classmates for clarification on any concepts you find challenging.
By thoroughly understanding neuron anatomy, the intricacies of action potentials and synaptic transmission, and applying this knowledge to practical scenarios like those potentially included in Exercise 13, you'll be well-equipped to succeed in your studies of this crucial area of neuroscience. Remember, consistent effort and a focus on understanding the underlying principles are key to mastering this complex but rewarding subject.
Latest Posts
Latest Posts
-
Ethical Approaches Prescribing An Ethical Approach
May 24, 2025
-
Do Swans Sing Before They Die
May 24, 2025
-
The Fault In Our Stars Sparknotes
May 24, 2025
-
The Kite Runner Summary Chapter 10
May 24, 2025
-
Bile Assists In The Chemical Digestion Of Triglycerides By
May 24, 2025
Related Post
Thank you for visiting our website which covers about Neuron Anatomy And Physiology Exercise 13 . We hope the information provided has been useful to you. Feel free to contact us if you have any questions or need further assistance. See you next time and don't miss to bookmark.