Motion Of Molecules Compared To Molecules
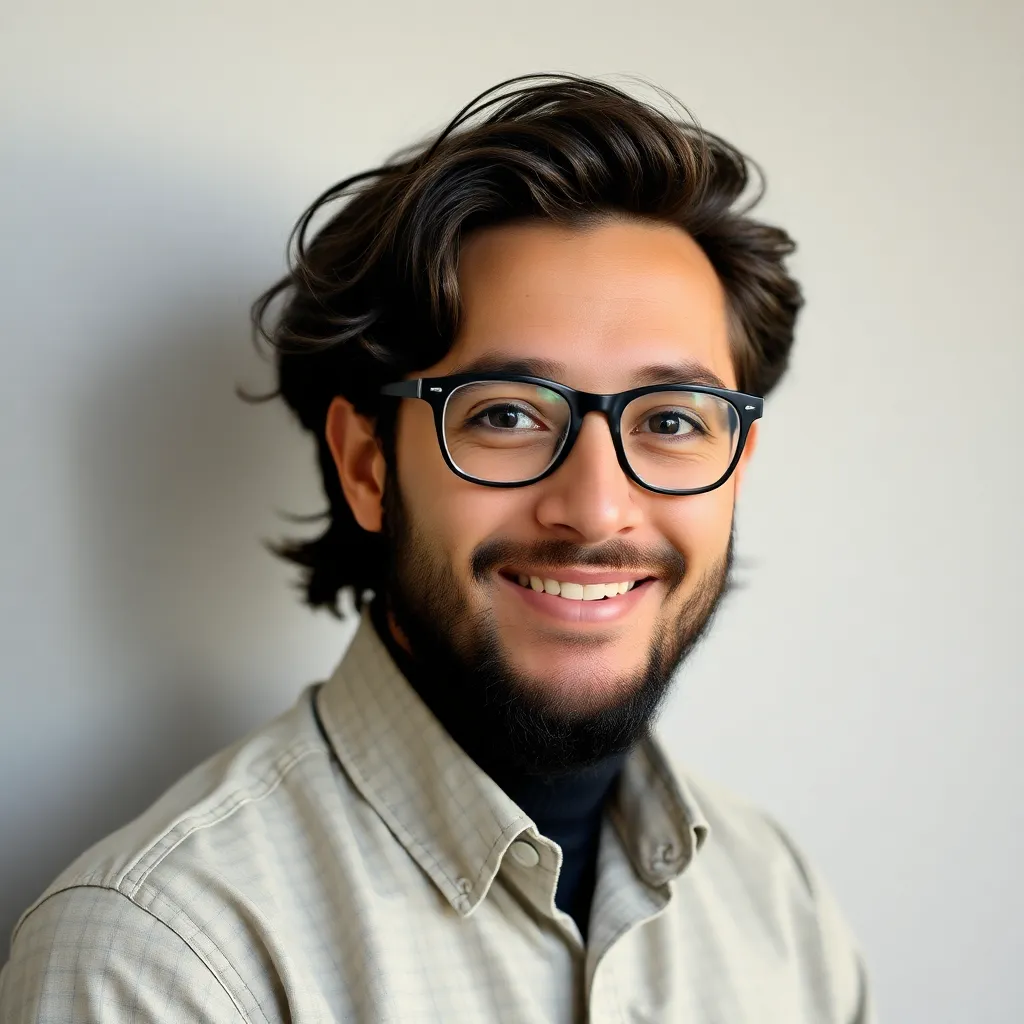
Juapaving
Apr 23, 2025 · 7 min read

Table of Contents
The Dance of Molecules: Comparing and Contrasting Molecular Motion
The world around us is a whirlwind of activity at the molecular level. From the simmering of a pot of water to the rustling of leaves in the wind, everything we experience is a manifestation of the constant motion of molecules. Understanding this motion, and the subtle differences in how various molecules move, is crucial to grasping the fundamentals of chemistry, physics, and even biology. This article delves deep into the fascinating realm of molecular motion, comparing and contrasting the dynamics of different types of molecules under varying conditions.
The Kinetic Molecular Theory: The Foundation of Molecular Motion
Before we dive into specific comparisons, it's essential to establish a foundational understanding of the kinetic molecular theory (KMT). This theory posits that all matter is composed of tiny particles (atoms and molecules) in constant, random motion. The energy of this motion is directly related to temperature; higher temperatures mean faster, more energetic molecular movement. This seemingly simple theory underpins a vast array of phenomena, from gas pressure to the rate of chemical reactions.
Key tenets of the KMT relevant to molecular motion:
- Constant motion: Molecules are perpetually in motion, whether they are solids, liquids, or gases. The nature of this motion, however, differs significantly between the phases of matter.
- Negligible intermolecular forces (in ideal gases): In ideal gases, the attractive and repulsive forces between molecules are assumed to be negligible compared to their kinetic energy. This simplifies calculations but is not entirely realistic for real-world gases.
- Elastic collisions: Collisions between molecules are considered elastic, meaning that kinetic energy is conserved. No energy is lost during these interactions.
- Average kinetic energy proportional to temperature: The average kinetic energy of molecules is directly proportional to the absolute temperature of the system.
Comparing Molecular Motion Across Phases of Matter
The most dramatic differences in molecular motion are observed when comparing the three primary phases of matter: solids, liquids, and gases.
Solids: Vibrational Motion Reigns Supreme
In solids, molecules are tightly packed together in a fixed, ordered arrangement. Their motion is largely restricted to vibrational movement around their equilibrium positions. They vibrate back and forth, but their movement is relatively small and localized. The strength of the intermolecular forces determines the amplitude of these vibrations. Stronger forces lead to smaller vibrations.
Examples: The molecules in a solid diamond vibrate intensely even at room temperature, but their overall position remains fixed within the crystal lattice structure. The rigidity and stability of solids are directly attributable to this limited, vibrational motion.
Liquids: Increased Freedom, Increased Motion
Liquids exhibit a much greater degree of molecular motion compared to solids. While molecules are still relatively close together, they are not fixed in place. They possess translational motion, meaning they can move from one location to another, albeit more slowly and with more frequent interactions than in gases. They also continue to exhibit vibrational and rotational motion. The fluidity of liquids is a direct consequence of this increased freedom of movement.
Examples: Water molecules in liquid water are constantly moving, colliding, and changing positions. The molecules are close enough for significant intermolecular interactions, such as hydrogen bonding, to affect their motion. These interactions influence the viscosity and other properties of the liquid.
Gases: The Realm of Unrestricted Motion
Gases represent the epitome of molecular freedom. In gases, molecules are widely dispersed and are subject to minimal intermolecular interactions. They exhibit all forms of motion: translational, rotational, and vibrational. Their movement is largely unrestricted, leading to rapid diffusion and expansion to fill their containers. The high kinetic energy of gas molecules overcomes the weak attractive forces between them.
Examples: Oxygen molecules in the air are constantly moving at high speeds, colliding with each other and with the walls of their container. The pressure exerted by a gas is a direct consequence of these frequent collisions. The randomness of their movement accounts for the ability of gases to readily mix.
Comparing Molecular Motion of Different Types of Molecules
The type of molecule also significantly impacts its motion. Several factors contribute to this:
Molecular Weight: The Mass Matters
Heavier molecules, with larger molecular weights, generally move more slowly than lighter molecules at the same temperature. This is because kinetic energy is shared among all the atoms in a molecule; a heavier molecule will have a lower velocity for the same kinetic energy.
Molecular Shape and Structure: Impacting Rotational and Translational Freedom
Molecular shape influences rotational and translational freedom. Linear molecules, such as carbon dioxide (CO2), rotate more easily than bulky, spherical molecules. Similarly, the shape can impact how easily a molecule translates through a medium. Long, chain-like molecules might experience more resistance than compact, spherical ones.
Intermolecular Forces: The Glue that Holds (or Doesn't Hold) Molecules Together
The strength of intermolecular forces (like hydrogen bonding, van der Waals forces, and dipole-dipole interactions) dramatically alters molecular motion. Stronger forces restrict movement, leading to slower diffusion rates, higher viscosities, and lower vapor pressures. Conversely, weaker forces allow for greater freedom of movement.
Examples:
- Water (H₂O): The strong hydrogen bonding in water significantly restricts the motion of its molecules compared to a gas like methane (CH₄) at the same temperature.
- Methane (CH₄): Methane's weak London dispersion forces allow for relatively unrestricted molecular motion.
- Large, complex biomolecules (proteins, DNA): The size and complex shapes of these molecules, combined with various intra- and intermolecular interactions, heavily restrict their movement in solution.
The Influence of Temperature and Pressure on Molecular Motion
Temperature and pressure are powerful external factors that directly influence molecular motion:
Temperature: The Kinetic Energy Booster
Increasing temperature directly increases the average kinetic energy of molecules. This leads to faster speeds and more energetic motion. The effect is particularly pronounced in gases where the increase in temperature translates directly into increased pressure if the volume is kept constant.
Pressure: Constraining Molecular Movement
Increasing pressure, especially in gases, forces molecules closer together, increasing the frequency of collisions. This can slightly reduce the average distance molecules travel between collisions but doesn’t necessarily reduce their average kinetic energy. The effect on molecular motion is more about the frequency and nature of the collisions than a change in velocity.
Applications of Understanding Molecular Motion
Understanding the principles of molecular motion has widespread applications across various scientific disciplines:
Chemistry: Reaction Rates and Equilibrium
Molecular motion is fundamental to chemical kinetics. The rate of a chemical reaction depends on the frequency and energy of collisions between reactant molecules. Higher temperatures, leading to faster molecular motion, generally result in faster reaction rates. Equilibrium constants also reflect the relative movement and interactions of molecules in different states.
Physics: Diffusion, Osmosis, and Thermodynamics
Molecular motion drives diffusion, the net movement of molecules from an area of high concentration to an area of low concentration. Osmosis, the movement of water across a semipermeable membrane, is also a direct consequence of water molecule motion. Thermodynamics, the study of energy and its transformations, relies heavily on understanding the kinetic energy of molecules.
Biology: Enzyme Activity and Cell Function
The activity of enzymes, biological catalysts, depends on the motion of both the enzyme and the substrate molecules. The binding of substrate to enzyme, a crucial step in catalysis, involves molecular interactions and motion. Many cellular processes, such as transport across cell membranes, also rely on the motion of molecules.
Conclusion: A Dynamic World at the Molecular Level
The dance of molecules is a constant, complex, and fascinating phenomenon. By comparing and contrasting the motion of different molecules under varying conditions, we gain a deeper appreciation for the underlying principles that govern the physical and chemical world. The kinetic molecular theory provides a robust framework for understanding this motion, and its implications extend across numerous scientific disciplines, highlighting the ubiquitous and fundamental nature of molecular movement. Further research into the intricacies of molecular dynamics continues to unveil deeper understandings, pushing the boundaries of scientific knowledge and its applications.
Latest Posts
Latest Posts
-
Examples Of A 1st Class Lever
Apr 23, 2025
-
What Is A Reactant In Biology
Apr 23, 2025
-
5 Letter Word That Starts With A D
Apr 23, 2025
-
What Is The Molecular Weight Of Oxygen
Apr 23, 2025
-
What Kingdom Do Humans Belong To
Apr 23, 2025
Related Post
Thank you for visiting our website which covers about Motion Of Molecules Compared To Molecules . We hope the information provided has been useful to you. Feel free to contact us if you have any questions or need further assistance. See you next time and don't miss to bookmark.