In Cellular Respiration Most Atp Molecules Are Produced By
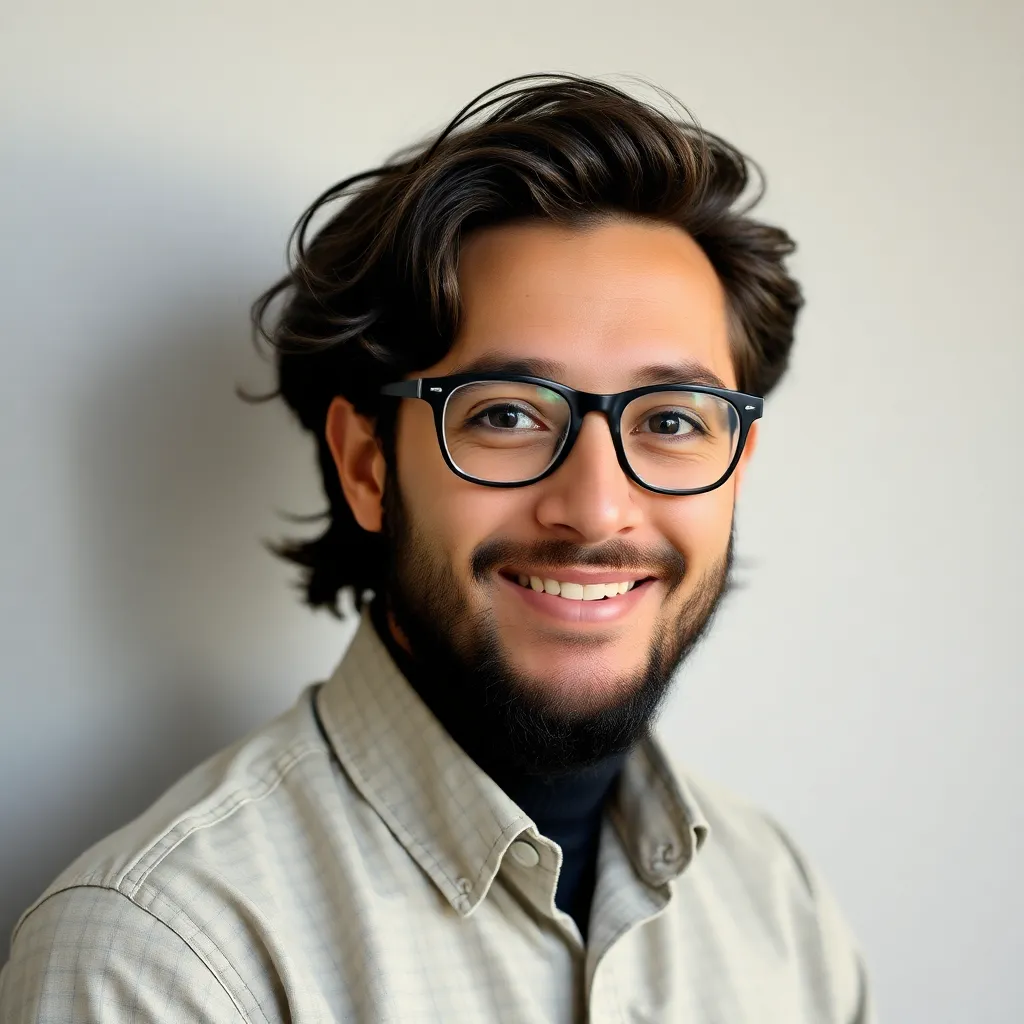
Juapaving
Apr 22, 2025 · 6 min read

Table of Contents
In Cellular Respiration, Most ATP Molecules Are Produced By Oxidative Phosphorylation
Cellular respiration is the process by which cells break down glucose and other organic molecules to produce ATP (adenosine triphosphate), the primary energy currency of the cell. While glycolysis and the citric acid cycle contribute to ATP production, the vast majority of ATP molecules are generated through a process called oxidative phosphorylation. Understanding this highly efficient energy-generating mechanism is crucial to grasping the intricacies of cellular metabolism. This article delves deep into oxidative phosphorylation, exploring its key components, the electron transport chain, chemiosmosis, and the factors influencing its efficiency.
The Role of Oxidative Phosphorylation in ATP Production
Oxidative phosphorylation is the final stage of cellular respiration, and it occurs in the mitochondria, often referred to as the "powerhouses" of the cell. This process elegantly couples the oxidation of electron carriers (NADH and FADH2) with the phosphorylation of ADP to ATP. The process can be broadly divided into two crucial components:
1. The Electron Transport Chain (ETC): A Cascade of Electron Transfer
The electron transport chain is a series of protein complexes embedded in the inner mitochondrial membrane. These complexes, numbered I-IV, facilitate the sequential transfer of electrons from NADH and FADH2, ultimately to oxygen. As electrons move down the chain, they lose energy, which is harnessed to pump protons (H+) from the mitochondrial matrix across the inner mitochondrial membrane into the intermembrane space. This creates a proton gradient, a crucial driving force for ATP synthesis.
-
Complex I (NADH dehydrogenase): Accepts electrons from NADH and transfers them to ubiquinone (Q), a mobile electron carrier. This transfer is coupled to proton pumping.
-
Complex II (Succinate dehydrogenase): Accepts electrons from FADH2 (produced during the citric acid cycle) and transfers them to ubiquinone. Unlike Complex I, it does not directly pump protons.
-
Complex III (Cytochrome bc1 complex): Receives electrons from ubiquinone and passes them to cytochrome c, another mobile electron carrier. This step also involves proton pumping.
-
Complex IV (Cytochrome c oxidase): The terminal electron acceptor complex, receiving electrons from cytochrome c and transferring them to molecular oxygen (O2), which is reduced to water (H2O). This step is also coupled to proton pumping.
The precise arrangement and interaction of these complexes within the inner mitochondrial membrane are critical for the efficient transfer of electrons and the generation of the proton gradient. Any disruption in this intricate structure can significantly impair ATP production.
2. Chemiosmosis: Harnessing the Proton Gradient for ATP Synthesis
The proton gradient established by the electron transport chain holds the key to ATP synthesis. This gradient represents a store of potential energy, and its dissipation drives the synthesis of ATP through a remarkable enzyme complex called ATP synthase.
ATP synthase, located in the inner mitochondrial membrane, acts as a molecular turbine. Protons flow down their concentration gradient from the intermembrane space back into the matrix through a channel within ATP synthase. This flow of protons causes a conformational change in the enzyme, driving the synthesis of ATP from ADP and inorganic phosphate (Pi). This process, known as chemiosmosis, is the essence of oxidative phosphorylation. The energy stored in the proton gradient is directly converted into the chemical energy of ATP.
The Efficiency of Oxidative Phosphorylation
Oxidative phosphorylation is remarkably efficient in converting the energy stored in NADH and FADH2 into ATP. Each NADH molecule contributes to the pumping of approximately 10 protons across the inner mitochondrial membrane, while each FADH2 molecule contributes to pumping approximately 6 protons. These protons drive the synthesis of approximately 2.5 ATP molecules per NADH and 1.5 ATP molecules per FADH2. The exact number can vary slightly depending on the efficiency of proton pumping and ATP synthase activity.
Factors Affecting Oxidative Phosphorylation Efficiency
Several factors can influence the efficiency of oxidative phosphorylation:
-
Oxygen availability: Oxygen is the final electron acceptor in the ETC. A lack of oxygen (hypoxia) halts electron transport, preventing proton pumping and ATP synthesis. This is why aerobic respiration is crucial for efficient energy production.
-
Substrate availability: The availability of NADH and FADH2, the electron carriers produced during glycolysis and the citric acid cycle, directly impacts the rate of oxidative phosphorylation. Sufficient supply of glucose and other metabolic fuels is essential.
-
Mitochondrial integrity: The structural integrity of mitochondria, particularly the inner mitochondrial membrane, is paramount for oxidative phosphorylation. Damage to the membrane can lead to reduced proton pumping and decreased ATP synthesis.
-
Uncoupling proteins: These proteins can disrupt the proton gradient by allowing protons to flow back into the matrix without passing through ATP synthase. While this reduces ATP production, it generates heat, a mechanism important in thermogenesis in brown adipose tissue.
-
Inhibitors and uncouplers: Various compounds can inhibit specific steps in the electron transport chain or uncouple proton pumping from ATP synthesis. These inhibitors and uncouplers can have significant consequences on cellular energy production.
Comparison to Other ATP Production Pathways
While oxidative phosphorylation generates the vast majority of ATP in cellular respiration, it's essential to understand the contributions of other pathways:
-
Glycolysis: This anaerobic process in the cytoplasm produces a small net yield of 2 ATP molecules per glucose molecule. Although less efficient than oxidative phosphorylation, it's crucial in situations with limited oxygen availability.
-
Citric acid cycle (Krebs cycle): This cycle in the mitochondrial matrix generates high-energy electron carriers (NADH and FADH2) and a small amount of ATP (2 ATP per glucose molecule). Its primary function is to feed electrons into the electron transport chain for oxidative phosphorylation.
Clinical Relevance and Disease Implications
Disruptions in oxidative phosphorylation can have severe health consequences, contributing to various diseases:
-
Mitochondrial diseases: These are a group of disorders arising from defects in mitochondrial function, often affecting energy-demanding tissues like the brain, heart, and muscles. Symptoms can range from mild to severe, depending on the severity and location of the mitochondrial dysfunction.
-
Neurodegenerative diseases: Impaired mitochondrial function is implicated in several neurodegenerative diseases, including Parkinson's and Alzheimer's diseases. The accumulation of reactive oxygen species (ROS) due to mitochondrial dysfunction can damage neuronal cells and contribute to neurodegeneration.
-
Cancer: Mitochondrial dysfunction can contribute to cancer development and progression. Cancer cells often exhibit altered metabolic pathways, including changes in oxidative phosphorylation, allowing them to survive and proliferate.
Conclusion: The Central Role of Oxidative Phosphorylation
Oxidative phosphorylation is the cornerstone of cellular energy production. Its remarkable efficiency in harnessing the energy from electron transfer and proton gradients makes it the primary source of ATP in aerobic organisms. Understanding its intricate mechanisms and the factors that regulate its efficiency is fundamental to comprehending cellular metabolism and its implications for health and disease. Disruptions in this process can have profound consequences, highlighting the critical role of oxidative phosphorylation in maintaining cellular homeostasis and overall organismal health. Further research into this crucial process is essential for developing effective therapeutic strategies for numerous diseases linked to mitochondrial dysfunction. The continued investigation into the intricacies of oxidative phosphorylation promises to unveil more about cellular energy metabolism and its wider implications for human health and disease.
Latest Posts
Latest Posts
-
What Does 2 1 Ratio Mean
Apr 22, 2025
-
Moment Of Inertia Of Spherical Shell
Apr 22, 2025
-
Difference Between Globular And Fibrous Protein
Apr 22, 2025
-
Which Is Greater 2 4 Or 2 3
Apr 22, 2025
-
Any Substance Dissolved In Water Is Called A
Apr 22, 2025
Related Post
Thank you for visiting our website which covers about In Cellular Respiration Most Atp Molecules Are Produced By . We hope the information provided has been useful to you. Feel free to contact us if you have any questions or need further assistance. See you next time and don't miss to bookmark.