In An Experiment Two Identical Rocks Are Simultaneously Thrown
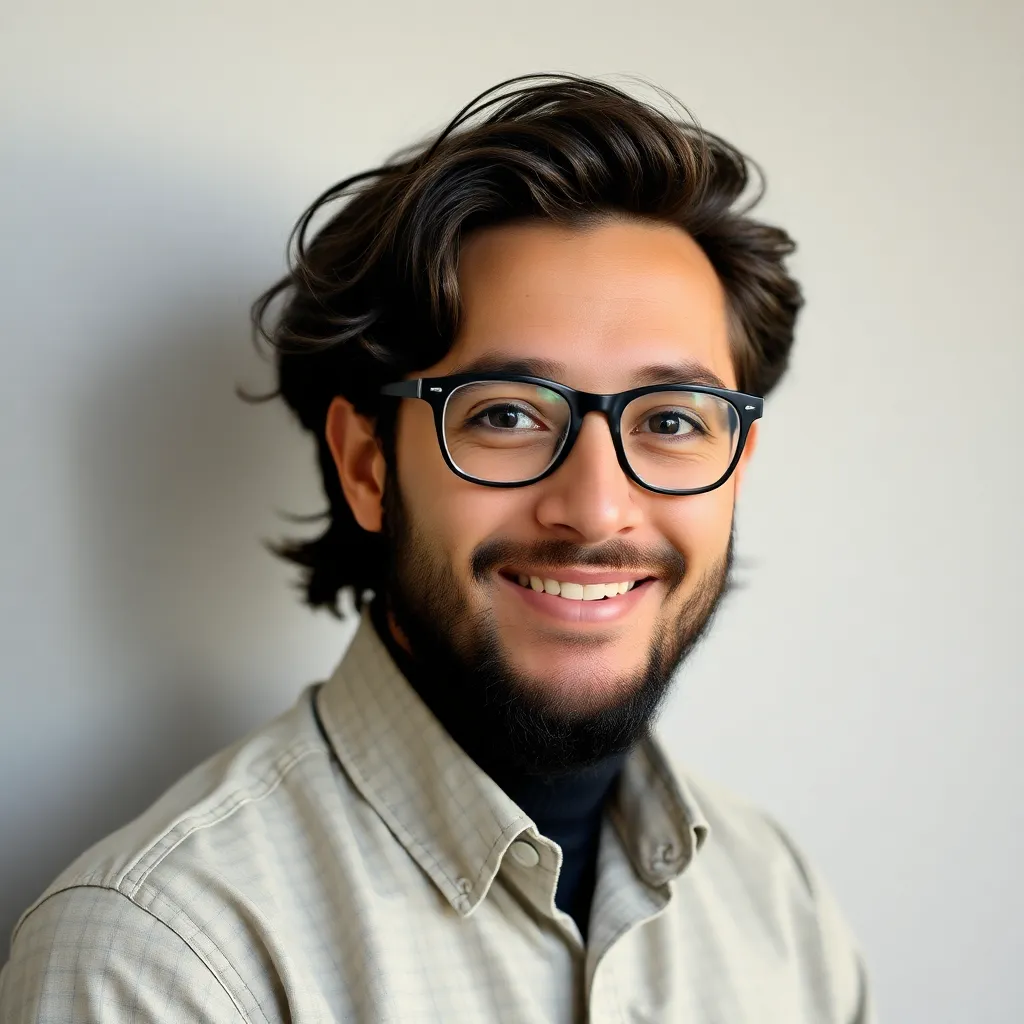
Juapaving
May 25, 2025 · 6 min read
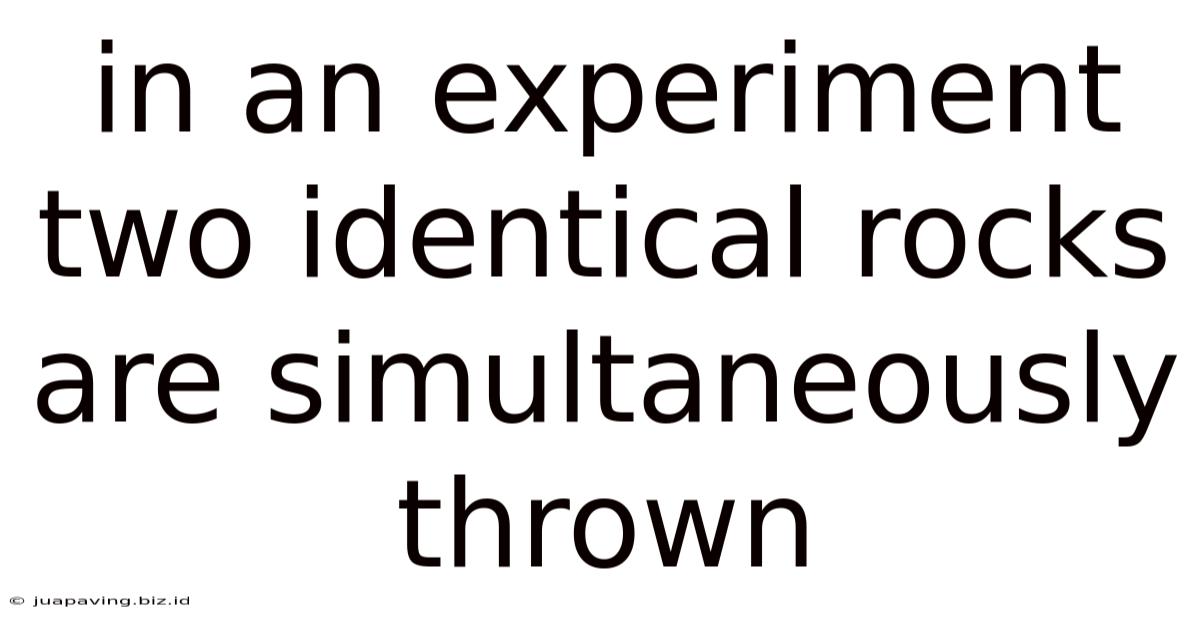
Table of Contents
In an Experiment, Two Identical Rocks are Simultaneously Thrown: Unveiling the Physics of Projectile Motion
The seemingly simple act of throwing two identical rocks simultaneously holds a wealth of fascinating physics within it. While it might appear straightforward, a closer examination reveals intricate principles governing projectile motion, air resistance, and the subtle nuances of identicality itself. This article delves deep into the experiment, exploring the theoretical underpinnings, practical considerations, and the surprising complexities that arise when gravity, air resistance, and initial conditions interact.
Understanding Projectile Motion: The Theoretical Framework
Before we even consider launching our rocks, we need a firm grasp on projectile motion. This is the motion of an object projected into the air, subject only to the force of gravity (and potentially air resistance). Several key factors determine a projectile's trajectory:
1. Initial Velocity:
This is the speed and direction at which the rock leaves the thrower's hand. It's crucial because it dictates the initial kinetic energy and directly influences the range and maximum height of the projectile. Even a slight difference in initial velocity between the two rocks will lead to different trajectories. This is where "identical" becomes a complex issue – perfectly identical initial velocities are extremely difficult to achieve in practice.
2. Angle of Projection:
The angle at which the rock is thrown relative to the horizontal plane significantly affects its path. A 45-degree angle maximizes the range (horizontal distance traveled) for a given initial velocity, neglecting air resistance. Any deviation from this angle reduces the range. Consistent launch angles are paramount for comparing the two rocks' trajectories.
3. Gravity:
This is the constant downward force acting on both rocks. It's responsible for the parabolic trajectory. Gravity's acceleration (approximately 9.8 m/s²) is uniform, meaning it doesn't change with the rock's velocity or position. This consistency makes it a predictable element in our calculations.
4. Air Resistance:
This is a force opposing the motion of the rock through the air. It's proportional to the rock's velocity and its cross-sectional area. Air resistance complicates projectile motion, making it non-parabolic. The faster the rock moves, the greater the air resistance. This is where identical rocks may still exhibit different behaviors. Minor imperfections in shape or surface texture can drastically alter air resistance, leading to discrepancies in trajectory.
The Experimental Setup: Controlling Variables for Accurate Results
To conduct a meaningful experiment, careful consideration must be given to the experimental setup. The goal is to minimize extraneous variables and isolate the impact of the factors mentioned above. This requires meticulous attention to detail:
1. Identical Rocks:
The quest for "identical" rocks is fraught with challenges. True identicality implies identical mass, shape, size, surface texture, and density. In practice, achieving this level of perfection is virtually impossible. Even slight variations in these properties can significantly influence the rocks' trajectory, especially when considering the effects of air resistance. The best we can aim for is rocks with minimal differences.
2. Consistent Throwing Technique:
The thrower’s technique must be standardized to minimize variations in initial velocity and launch angle. This might involve using a simple throwing device to ensure consistent release and minimize human error. Repeated practice to develop a consistent throwing motion is essential.
3. Controlled Environment:
The experiment should ideally be conducted in a controlled environment with minimal wind. Wind introduces an unpredictable force affecting the trajectory, making it difficult to isolate the effects of gravity and air resistance. An indoor setting with minimal air currents is preferred.
4. Measurement Tools:
Accurate measurements of launch angle, initial velocity, and the rocks' trajectories are crucial. Tools like high-speed cameras, motion sensors, or even careful manual measurements with a stopwatch and measuring tape can be utilized, depending on the desired level of precision.
Analyzing the Results: Interpreting the Data
Once the experiment is conducted, the data gathered needs careful analysis. This involves comparing the trajectories of the two rocks, noting any differences, and attempting to explain these discrepancies.
1. Range and Maximum Height:
Comparing the horizontal range (distance traveled) and maximum height reached by each rock is the first step. Any substantial difference indicates variations in initial velocity, launch angle, or air resistance. The degree of difference provides insight into the magnitude of these variations.
2. Time of Flight:
The time each rock spends in the air can also be a valuable indicator. Differences here might reflect disparities in initial velocity or the effect of air resistance. A rock experiencing higher air resistance will generally have a shorter time of flight.
3. Trajectory Shape:
While ideally, the trajectory should be a parabola (neglecting air resistance), any deviations from this shape point to the influence of air resistance and the subtle differences between the rocks. Asymmetrical trajectories are often a clear indicator of non-uniform air resistance due to shape irregularities.
4. Statistical Analysis:
For increased accuracy, repeating the experiment multiple times and performing statistical analysis on the collected data is recommended. This helps to minimize the impact of random errors and reveal any systematic differences between the rocks' trajectories.
Beyond Identicality: Exploring the Role of Imperfections
The experiment highlights the difficulty of creating truly identical objects in the real world. Even minor imperfections can significantly alter the behavior of objects in motion. This underscores the importance of understanding and accounting for such imperfections in physics experiments and real-world applications.
1. Surface Roughness:
Variations in surface texture affect the interaction with air molecules, influencing the drag force. A rougher surface generally experiences greater air resistance than a smooth surface.
2. Shape Irregularities:
Even minuscule variations in shape can cause differences in air resistance and therefore trajectory. This is why aerodynamic design is so critical in fields such as aerospace engineering.
3. Density Variations:
Differences in density, even if minor, affect the overall mass and inertial properties of the rock, impacting the response to gravitational forces.
4. Internal Structure:
Internal inconsistencies in rock composition can affect the overall mass distribution and potentially alter its flight characteristics.
Real-World Applications: Relevance Beyond the Experiment
The principles revealed by this seemingly simple experiment have wide-ranging applications in various fields:
1. Ballistics:
Understanding projectile motion is crucial in ballistics, which involves the science of launching projectiles. Accurate trajectory prediction is essential for artillery, firearms, and even sports like archery and golf.
2. Aerospace Engineering:
Designing aircraft and spacecraft relies heavily on understanding air resistance and projectile motion. Minimizing drag is crucial for fuel efficiency and performance.
3. Meteorology:
Tracking weather patterns and understanding the trajectory of weather systems involves considering the effects of gravity, wind, and air resistance on atmospheric phenomena.
4. Sports Science:
Analyzing the trajectory of balls in sports like baseball, basketball, and soccer involves the same principles of projectile motion, assisting in improving athletic performance and technique.
Conclusion: The Unfolding Story of Imperfect Identicality
The experiment of simultaneously throwing two "identical" rocks serves as a powerful illustration of the complexities of projectile motion. While the theoretical framework is relatively straightforward, the practical implementation exposes the challenges of achieving perfect identicality and the significant impact of seemingly minor imperfections. The discrepancies observed highlight the importance of carefully controlled experimental conditions, precise measurement techniques, and the profound influence of air resistance. The insights gained from this simple experiment translate to a deeper understanding of fundamental physical principles and their far-reaching implications in various fields of science and engineering. It underscores the fact that even in seemingly simple scenarios, a thorough understanding of the underlying physics requires meticulous attention to detail and a nuanced appreciation for the influence of even subtle variations.
Latest Posts
Latest Posts
-
Shortest Sentence In The English Language
May 25, 2025
-
Hairlike Processes That Project From Epithelial Cells Are Called
May 25, 2025
-
Amigos De Todas Partes Vhl Answers
May 25, 2025
-
A Christmas Carol Stave 5 Summary
May 25, 2025
-
Closed Innovation Is Associated With Which Organizational Inertia Problem
May 25, 2025
Related Post
Thank you for visiting our website which covers about In An Experiment Two Identical Rocks Are Simultaneously Thrown . We hope the information provided has been useful to you. Feel free to contact us if you have any questions or need further assistance. See you next time and don't miss to bookmark.