How Do C4 Plants Minimize Photorespiration
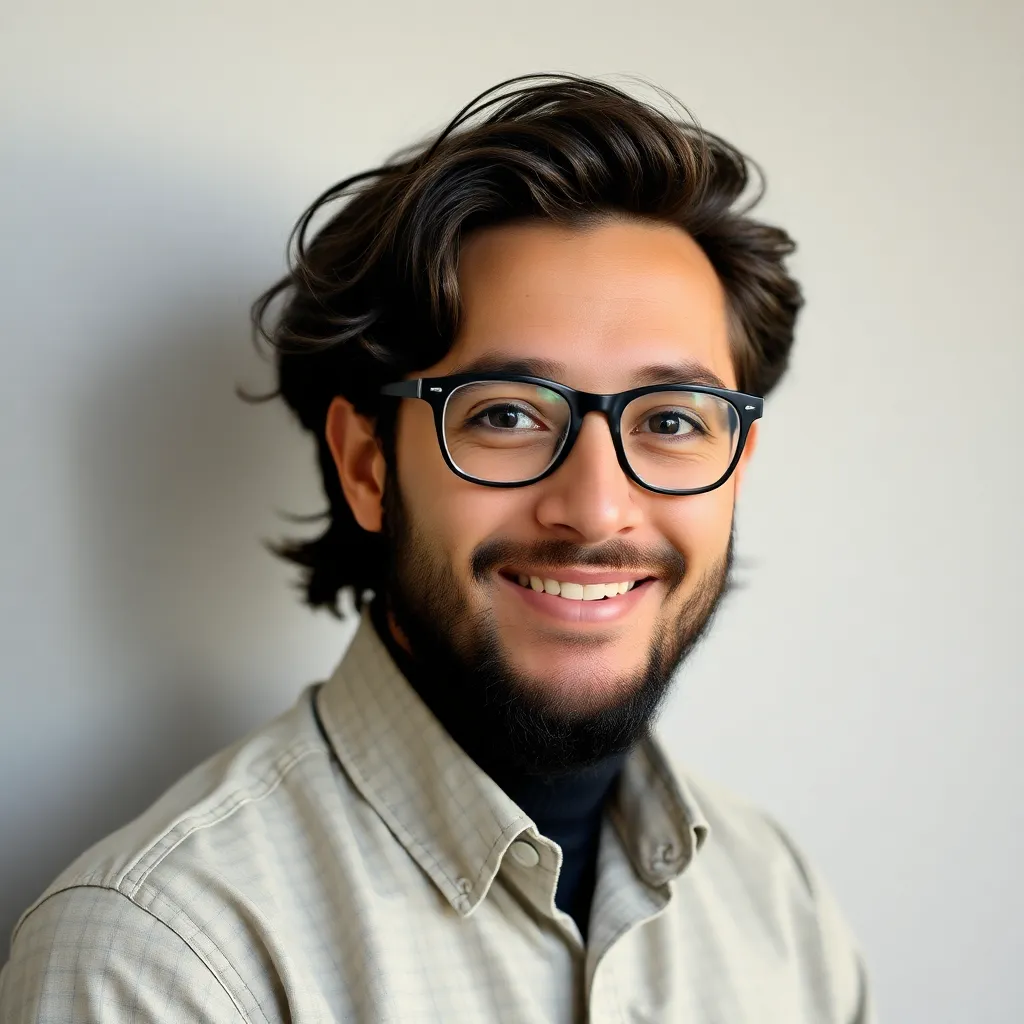
Juapaving
May 09, 2025 · 6 min read
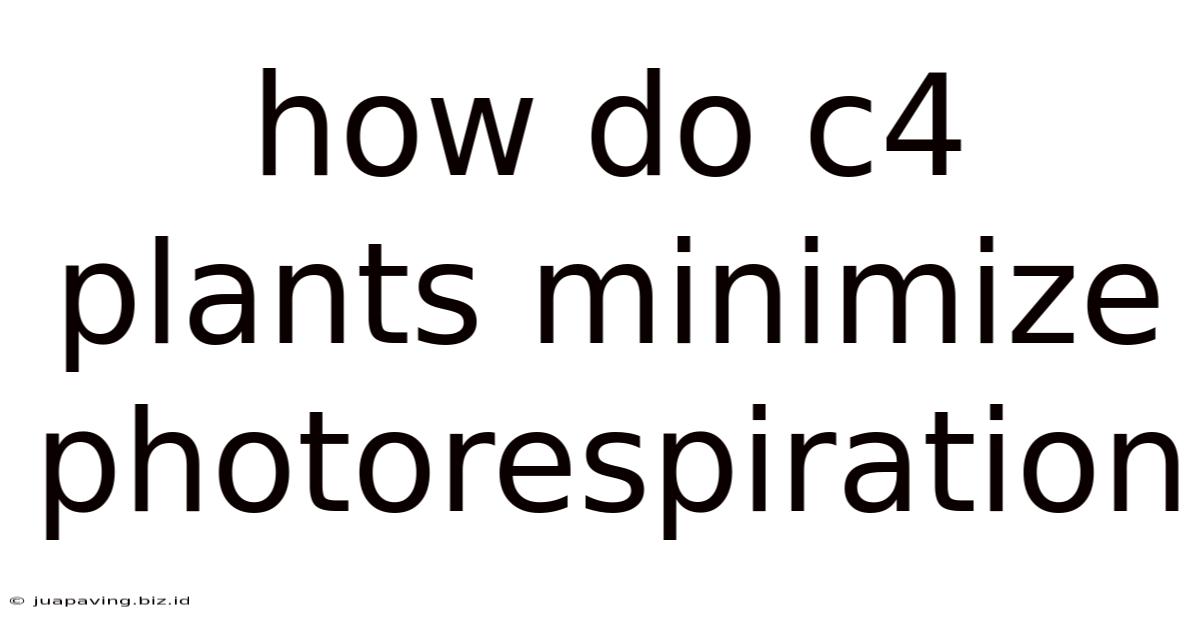
Table of Contents
How C4 Plants Minimize Photorespiration: A Deep Dive into Photosynthetic Efficiency
Photorespiration, a metabolic process that competes with photosynthesis, significantly reduces the efficiency of carbon fixation in many plants. While seemingly counterintuitive – respiration occurring in the presence of light – it's a significant drain on energy and resources. However, certain plants, known as C4 plants, have evolved ingenious mechanisms to minimize photorespiration and enhance their photosynthetic efficiency, particularly in hot, dry, and sunny environments. This article delves deep into the intricacies of how C4 plants achieve this remarkable feat, exploring the biochemical pathways, anatomical adaptations, and ecological implications.
Understanding Photorespiration: The Enemy of Photosynthesis
Before examining the solutions employed by C4 plants, let's establish a firm understanding of the problem itself. Photorespiration occurs when the enzyme RuBisCO, responsible for fixing carbon dioxide (CO2) in the Calvin cycle, mistakenly binds with oxygen (O2) instead. This oxygenation reaction leads to a series of reactions that consume energy and release previously fixed carbon as CO2, effectively undoing the work of photosynthesis.
The Role of RuBisCO and its Dual Functionality
RuBisCO's dual functionality – its ability to catalyze both carboxylation (adding CO2) and oxygenation (adding O2) – is a central aspect of this problem. While RuBisCO's affinity for CO2 is higher, its activity is strongly influenced by the relative concentrations of CO2 and O2 within the chloroplast. In hot and dry conditions, stomata close to conserve water, leading to a decrease in CO2 concentration and an increase in O2 concentration inside the leaf. This shift in the gas ratio favors oxygenation over carboxylation, boosting photorespiration.
The Cost of Photorespiration: Energy and Carbon Loss
The consequences of photorespiration are far-reaching. The process consumes ATP and NADPH, valuable energy molecules produced during the light-dependent reactions of photosynthesis. Moreover, it releases fixed carbon as CO2, diminishing the net gain of carbohydrates crucial for plant growth and development. The loss of fixed carbon reduces overall photosynthetic efficiency, especially under conditions that favor oxygenation, such as high temperatures, high light intensity, and low CO2 concentrations.
The C4 Solution: Spatial Segregation of Carbon Fixation
C4 plants have evolved a remarkable strategy to circumvent the limitations imposed by RuBisCO's dual functionality and minimize photorespiration. This strategy involves the spatial separation of initial CO2 fixation from the Calvin cycle. Instead of occurring solely within the mesophyll cells, as in C3 plants, the initial carbon fixation in C4 plants takes place in specialized mesophyll cells, while the Calvin cycle operates exclusively in bundle sheath cells.
The Hatch-Slack Pathway: A Two-Stage Carbon Fixation Process
The key to C4 photosynthesis lies in the Hatch-Slack pathway, a four-carbon pathway that precedes the Calvin cycle. This pathway involves two key enzymes:
-
PEP carboxylase (PEPC): This enzyme, located in the mesophyll cells, has a high affinity for CO2 and a very low affinity for O2. It catalyzes the initial fixation of CO2 by combining it with phosphoenolpyruvate (PEP), forming oxaloacetate (a four-carbon compound), hence the name C4.
-
RuBisCO: This enzyme, situated within the bundle sheath cells, continues its role in the Calvin cycle, but now operates in an environment enriched in CO2.
The Process: From Mesophyll to Bundle Sheath
The process unfolds as follows:
-
CO2 uptake: CO2 enters the leaf through stomata and diffuses into the mesophyll cells.
-
Initial fixation: PEPC fixes CO2 into PEP, creating oxaloacetate. This reaction is highly efficient and avoids oxygenation because of PEPC’s preference for CO2.
-
Conversion and transport: Oxaloacetate is then converted to malate or aspartate, four-carbon compounds that are transported to the bundle sheath cells.
-
Decarboxylation: Inside the bundle sheath cells, malate or aspartate is decarboxylated, releasing CO2. This creates a high concentration of CO2 around RuBisCO.
-
Calvin cycle: The released CO2 is now efficiently fixed by RuBisCO in the Calvin cycle, producing carbohydrates.
-
Pyruvate recycling: The three-carbon byproduct of decarboxylation (pyruvate) is transported back to the mesophyll cells, where it is converted back to PEP, completing the cycle.
Anatomical Adaptations: Kranz Anatomy
The spatial separation of carbon fixation is facilitated by a specialized leaf anatomy called Kranz anatomy ("wreath" in German). This anatomy features:
-
Bundle sheath cells: These cells are arranged in a ring around the vascular bundles (veins) and are densely packed with chloroplasts. The thick-walled bundle sheath cells create a barrier that helps maintain a high CO2 concentration around RuBisCO.
-
Mesophyll cells: These cells are located between the epidermis and the bundle sheath cells and are the primary sites of CO2 uptake and the initial fixation by PEPC.
This distinct arrangement ensures the efficient transport of four-carbon compounds from the mesophyll cells to the bundle sheath cells, maximizing CO2 concentration around RuBisCO and minimizing photorespiration.
Ecological Advantages: Thriving in Harsh Environments
The C4 photosynthetic pathway confers several significant ecological advantages, particularly in environments where photorespiration is a major constraint on photosynthetic efficiency:
-
High temperatures: C4 plants are better adapted to high temperatures than C3 plants because PEPC's activity is less sensitive to temperature than RuBisCO's. This allows them to maintain high photosynthetic rates even under hot and sunny conditions.
-
Water stress: The efficient CO2-concentrating mechanism of C4 plants allows them to maintain high photosynthetic rates with lower stomatal conductance, reducing water loss through transpiration. This is crucial for survival in dry environments.
-
Nitrogen use efficiency: C4 plants often exhibit higher nitrogen use efficiency than C3 plants. While they invest more nitrogen in PEPC, the reduction in photorespiration and the efficient use of RuBisCO compensates for this.
-
Competitive advantage: The increased photosynthetic efficiency of C4 plants gives them a competitive advantage over C3 plants in many habitats, particularly those characterized by high light intensity, high temperatures, and water scarcity.
Diversity of C4 Plants: Variations on a Theme
While the fundamental principles of C4 photosynthesis remain consistent, there is substantial diversity in the specific biochemical pathways and anatomical features exhibited by different C4 species. This diversity reflects the evolutionary convergence on a common solution in response to similar environmental pressures. Three main subtypes exist, differing in the specific four-carbon compound transported from mesophyll to bundle sheath cells: NADP-ME, NAD-ME, and PEP-CK. These differences affect the energetic cost and efficiency of the C4 cycle, reflecting adaptation to different environmental conditions.
The Future of C4 Research: Engineering Enhanced Photosynthesis
Given the significant advantages of C4 photosynthesis, scientists are actively exploring the possibility of engineering C4 characteristics into C3 crops. This could lead to significant improvements in crop yield and resource use efficiency, thereby enhancing global food security. This research faces significant challenges, including the complex genetic and metabolic engineering required to replicate the intricate C4 pathway and Kranz anatomy. However, the potential benefits are substantial, making this a significant area of ongoing research and development.
Conclusion: A Triumph of Evolutionary Adaptation
C4 photosynthesis stands as a remarkable example of evolutionary adaptation. By spatially separating CO2 fixation and the Calvin cycle and employing specialized enzymes and leaf anatomy, C4 plants have effectively minimized the negative impacts of photorespiration. This enhanced photosynthetic efficiency provides them with a significant competitive advantage in hot, dry, and sunny environments, highlighting the power of natural selection to optimize metabolic pathways for survival and success. The study of C4 plants offers valuable insights into the intricate mechanisms of photosynthesis and holds significant promise for enhancing crop productivity and addressing global food security challenges. The ongoing research into understanding and manipulating C4 pathways promises to continue unlocking the secrets of efficient photosynthesis and its potential to revolutionize agriculture.
Latest Posts
Latest Posts
-
Label The Parts Of The Male Reproductive System
May 10, 2025
-
A Lens Is Used In A Magnifying Glass
May 10, 2025
-
How To Calculate The Ionization Energy Of Hydrogen
May 10, 2025
-
Are There Ribosomes In Prokaryotic Cells
May 10, 2025
-
5 Letter Words Beginning With Ten
May 10, 2025
Related Post
Thank you for visiting our website which covers about How Do C4 Plants Minimize Photorespiration . We hope the information provided has been useful to you. Feel free to contact us if you have any questions or need further assistance. See you next time and don't miss to bookmark.