Explain The Sliding Filament Theory Of Muscle Contraction
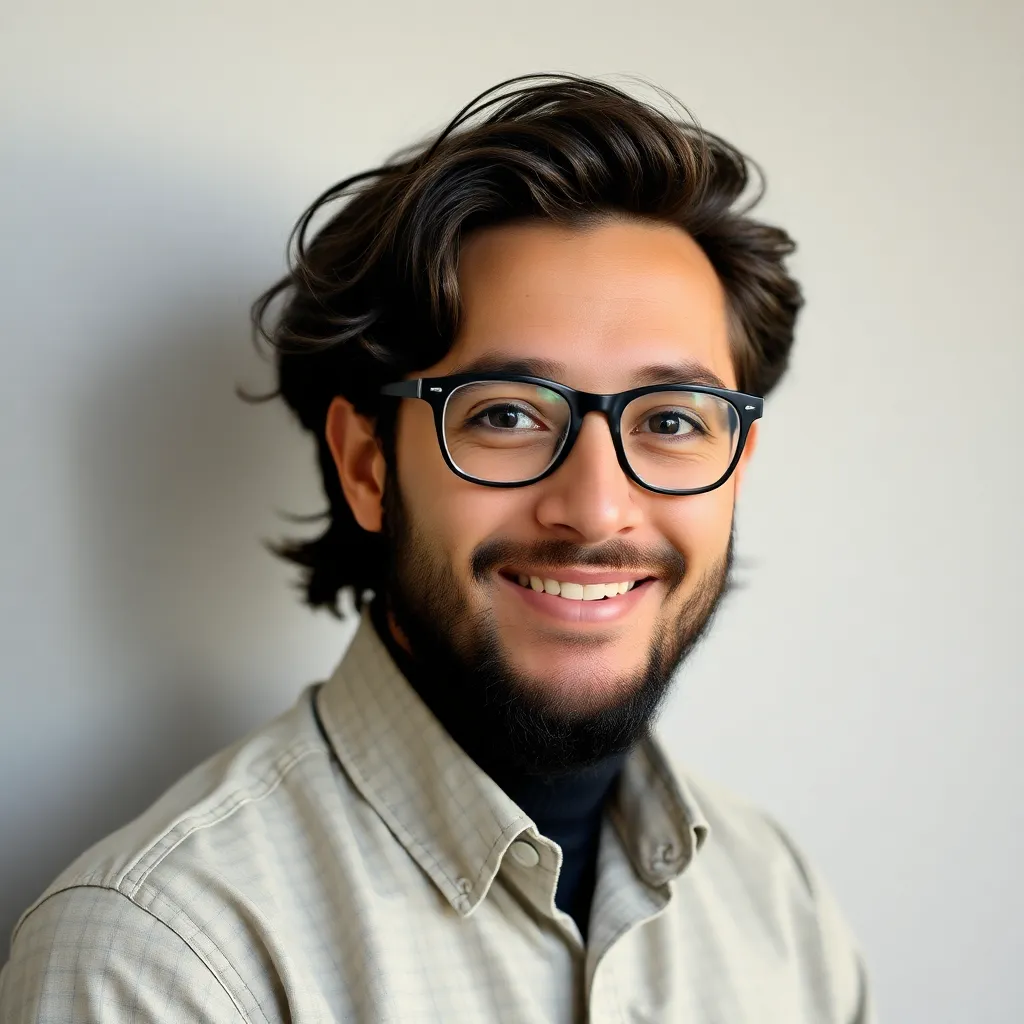
Juapaving
Apr 27, 2025 · 6 min read

Table of Contents
The Sliding Filament Theory: Unraveling the Mechanics of Muscle Contraction
The human body is a marvel of engineering, capable of feats of strength, agility, and endurance. At the heart of this capability lies the intricate process of muscle contraction, a phenomenon explained by the sliding filament theory. This theory, a cornerstone of modern physiology, details the molecular mechanisms that allow muscles to generate force and movement. Understanding this theory is crucial for comprehending not only athletic performance but also various medical conditions affecting muscle function. This comprehensive article will delve deep into the sliding filament theory, exploring its components, the role of key players like actin and myosin, the intricacies of the neuromuscular junction, and the energetic demands of muscle contraction. We will also touch upon the different types of muscle contractions and the factors affecting muscle performance.
The Molecular Players: Actin and Myosin
The sliding filament theory centers around the interaction of two primary proteins: actin and myosin. These proteins are organized within the muscle fiber in highly structured units called sarcomeres. Think of a sarcomere as the fundamental unit of muscle contraction – the smallest functional unit responsible for generating force.
Actin Filaments: The Thin Filaments
Actin filaments, also known as thin filaments, are composed primarily of the globular protein G-actin, which polymerizes to form long, fibrous F-actin strands. These F-actin strands are twisted together to create a double helix structure. Associated with the actin filaments are two other important proteins:
- Tropomyosin: A long, fibrous protein that wraps around the F-actin strands. In a relaxed muscle, tropomyosin physically blocks the myosin-binding sites on actin, preventing contraction.
- Troponin: A complex of three proteins (troponin T, troponin I, and troponin C) that regulates the position of tropomyosin. Troponin C has a high affinity for calcium ions (Ca²⁺).
Myosin Filaments: The Thick Filaments
Myosin filaments, also known as thick filaments, are composed of numerous myosin molecules. Each myosin molecule has a head region and a tail region. The myosin heads possess ATPase activity, meaning they can hydrolyze ATP (adenosine triphosphate) to release energy, which is essential for muscle contraction. The myosin heads also contain binding sites for both actin and ATP. The myosin molecules are arranged in a bipolar fashion within the filament, with the heads projecting outward towards the surrounding actin filaments.
The Neuromuscular Junction: Initiating the Contraction
Muscle contraction doesn't happen spontaneously. It's initiated by a signal from the nervous system at the neuromuscular junction. This specialized synapse transmits the nerve impulse to the muscle fiber, triggering a cascade of events leading to contraction.
The Role of Acetylcholine
The process begins with the release of acetylcholine, a neurotransmitter, from the motor neuron's axon terminal. Acetylcholine diffuses across the synaptic cleft and binds to receptors on the muscle fiber's membrane (sarcolemma). This binding depolarizes the sarcolemma, generating an action potential that propagates along the muscle fiber.
The Sarcoplasmic Reticulum and Calcium Release
The action potential then travels down specialized structures called T-tubules, which invaginate the sarcolemma and are in close proximity to the sarcoplasmic reticulum (SR). The SR is an intracellular calcium store. The action potential triggers the release of Ca²⁺ from the SR into the sarcoplasm (cytoplasm of the muscle cell). This calcium ion surge is the crucial trigger for muscle contraction.
The Cross-Bridge Cycle: The Sliding Mechanism
The increased sarcoplasmic Ca²⁺ concentration binds to troponin C. This binding causes a conformational change in the troponin-tropomyosin complex, moving tropomyosin away from the myosin-binding sites on actin. This exposes the binding sites, allowing the myosin heads to interact with actin.
The interaction between actin and myosin involves a cyclical process known as the cross-bridge cycle:
- Attachment: The myosin head, energized by ATP hydrolysis, binds to the exposed actin binding site, forming a cross-bridge.
- Power Stroke: The myosin head pivots, pulling the actin filament towards the center of the sarcomere. This is the power stroke, generating the force of muscle contraction. ADP and inorganic phosphate (Pi) are released during this step.
- Detachment: A new ATP molecule binds to the myosin head, causing it to detach from the actin filament.
- Reactivation: ATP is hydrolyzed, energizing the myosin head and returning it to its high-energy conformation, ready to initiate another cycle.
This cycle repeats multiple times as long as Ca²⁺ remains bound to troponin and ATP is available. The coordinated action of numerous myosin heads along the length of the sarcomere causes the actin and myosin filaments to slide past each other, resulting in sarcomere shortening and muscle contraction.
Relaxation: Turning Off the Contraction
Muscle relaxation occurs when the nerve impulse ceases. This leads to a decrease in the release of acetylcholine at the neuromuscular junction. Consequently, the sarcolemma repolarizes, and the SR actively pumps Ca²⁺ back into its lumen. As the sarcoplasmic Ca²⁺ concentration falls, Ca²⁺ detaches from troponin C. Tropomyosin once again blocks the myosin-binding sites on actin, preventing further cross-bridge cycling, and the muscle relaxes.
Types of Muscle Contractions
Muscle contractions can be categorized into several types based on their length and tension changes:
- Isotonic contractions: These contractions involve changes in muscle length while maintaining a constant tension. Examples include lifting a weight (concentric contraction – muscle shortens) or lowering a weight (eccentric contraction – muscle lengthens).
- Isometric contractions: In these contractions, muscle length remains constant, but tension increases. An example is pushing against an immovable object.
- Auxotonic contractions: These are a combination of isotonic and isometric contractions, where both length and tension change during contraction.
Factors Affecting Muscle Performance
Several factors influence the strength and duration of muscle contraction:
- Number of motor units recruited: The more motor units activated, the stronger the contraction.
- Frequency of stimulation: Increasing the frequency of nerve impulses leads to summation of contractions, resulting in a stronger and more sustained contraction (tetanus).
- Length-tension relationship: Muscle fibers have an optimal length for generating maximum force. Too short or too long, and the force generated is reduced.
- Muscle fatigue: Prolonged or intense muscle activity leads to fatigue, a decline in force production due to factors like depletion of energy stores (ATP), accumulation of metabolic byproducts, and changes in ion concentrations.
Clinical Significance of the Sliding Filament Theory
Understanding the sliding filament theory has significant clinical implications. Many muscle disorders, such as muscular dystrophy, myasthenia gravis, and various myopathies, involve defects in the components of the contractile machinery or the neuromuscular junction. Knowledge of these mechanisms is essential for developing diagnostic tools and therapeutic strategies for these conditions.
Conclusion: A Dynamic Process
The sliding filament theory provides a comprehensive explanation of how muscles contract, a process elegantly orchestrated by the interaction of actin and myosin, regulated by calcium ions, and driven by ATP hydrolysis. It highlights the intricate interplay between the nervous system and the muscular system, underscoring the body's remarkable ability to generate movement and perform various functions. Further research continues to refine our understanding of the complexities of muscle contraction, paving the way for advancements in treating muscle disorders and enhancing athletic performance. The sliding filament theory remains a fundamental concept in physiology, and its understanding is essential for anyone interested in the workings of the human body.
Latest Posts
Latest Posts
-
Five Letter Word Ending With E N
Apr 27, 2025
-
What Type Of Account Is Sales
Apr 27, 2025
-
Which State Of Matter Has The Most Energy
Apr 27, 2025
-
Which Is Bigger 1 2 Or 1 4
Apr 27, 2025
-
Is C A Metal Or Nonmetal
Apr 27, 2025
Related Post
Thank you for visiting our website which covers about Explain The Sliding Filament Theory Of Muscle Contraction . We hope the information provided has been useful to you. Feel free to contact us if you have any questions or need further assistance. See you next time and don't miss to bookmark.