Experiment 24 A Rate Law And Activation Energy
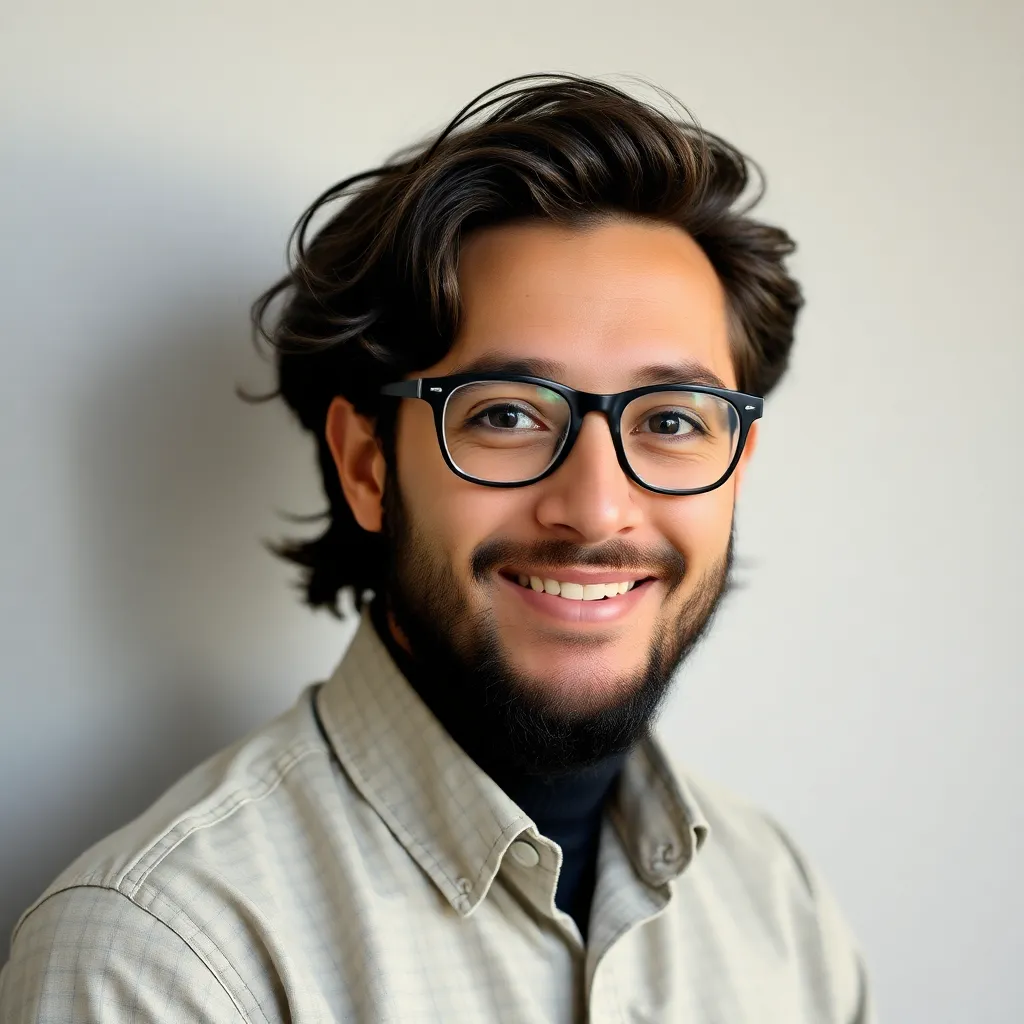
Juapaving
May 23, 2025 · 5 min read
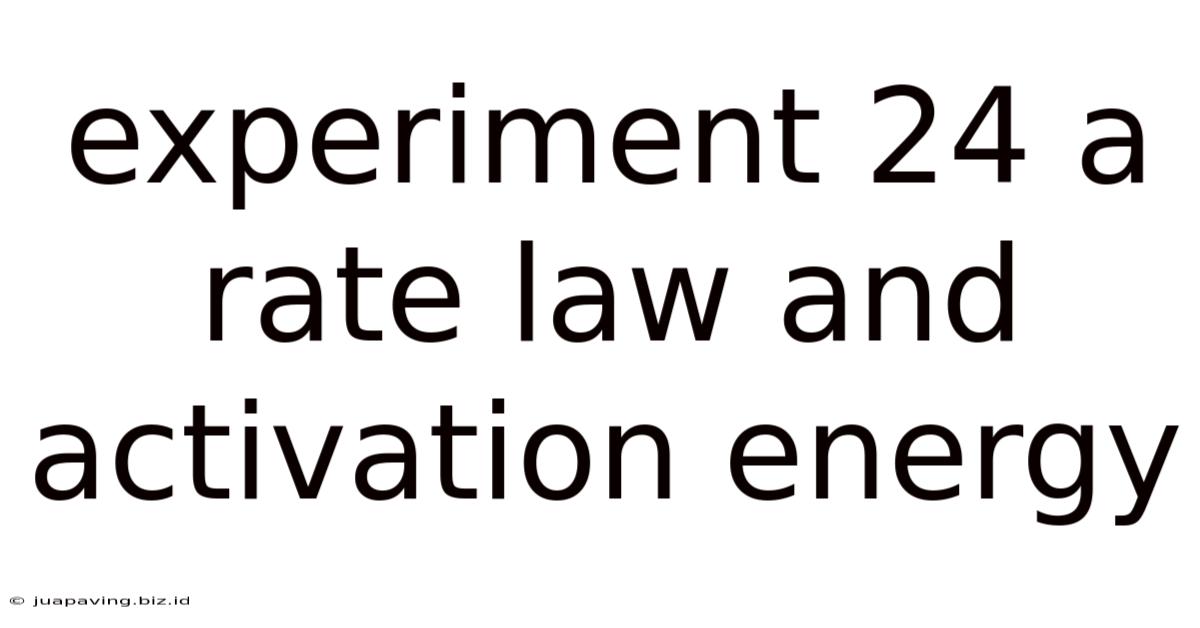
Table of Contents
Experiment 24: Unveiling the Secrets of Rate Laws and Activation Energy
Understanding chemical kinetics is fundamental to numerous scientific disciplines, from industrial chemistry to biochemistry. This article delves into the intricacies of Experiment 24, a common laboratory exercise designed to determine the rate law and activation energy of a chemical reaction. We will explore the theoretical underpinnings, practical procedures, data analysis techniques, and potential sources of error, offering a comprehensive guide for students and enthusiasts alike.
Understanding Rate Laws and Activation Energy
Before embarking on the experimental aspects, let's solidify our understanding of the core concepts:
Rate Laws: Quantifying Reaction Speed
The rate law of a reaction describes the relationship between the reaction rate and the concentrations of reactants. It generally takes the form:
Rate = k[A]<sup>m</sup>[B]<sup>n</sup>
Where:
- Rate: The speed at which the reaction proceeds (often measured in M/s or mol/L·s).
- k: The rate constant, a temperature-dependent proportionality constant.
- [A] and [B]: The concentrations of reactants A and B (in molarity).
- m and n: The reaction orders with respect to reactants A and B, respectively. These are experimentally determined exponents, not necessarily equal to the stoichiometric coefficients.
Activation Energy: The Energy Barrier
The activation energy (Ea) represents the minimum energy required for reactants to overcome the energy barrier and transform into products. It's a crucial factor influencing reaction rate; a higher activation energy implies a slower reaction at a given temperature. The relationship between rate constant (k) and activation energy is elegantly described by the Arrhenius equation:
k = Ae<sup>-Ea/RT</sup>
Where:
- A: The pre-exponential factor (frequency factor), representing the frequency of collisions with the correct orientation.
- Ea: Activation energy (in J/mol or kJ/mol).
- R: The ideal gas constant (8.314 J/mol·K).
- T: The absolute temperature (in Kelvin).
Experiment 24: A Detailed Procedure
Experiment 24 typically involves a reaction whose rate can be conveniently monitored, often using spectrophotometry or titration. A common example involves the reaction between crystal violet (a colored dye) and sodium hydroxide (a strong base). The fading of the crystal violet's color over time is readily monitored using a spectrophotometer. Here's a generalized procedure:
Materials and Equipment:
- Reactants: Precisely measured quantities of the reactants (e.g., crystal violet and NaOH).
- Spectrophotometer: To measure the absorbance of the reaction mixture at a specific wavelength.
- Cuvettes: For holding the reaction mixture in the spectrophotometer.
- Timer: To accurately record reaction times.
- Thermometer: To measure and control the reaction temperature.
- Water bath (optional): To maintain a constant temperature during the experiment.
- Pipettes and volumetric flasks: For precise volume measurements.
Experimental Steps:
- Preparation of Solutions: Prepare stock solutions of the reactants with known concentrations.
- Temperature Control: Maintain a constant temperature throughout the experiment using a water bath or by ensuring the reaction occurs in a thermally stable environment.
- Mixing Reactants: Quickly mix the reactants in a cuvette, initiating the reaction. Start the timer simultaneously.
- Spectrophotometric Measurements: Record the absorbance of the reaction mixture at regular intervals (e.g., every 30 seconds or 1 minute). The chosen wavelength should correspond to the maximum absorbance of the reactant (e.g., the crystal violet).
- Data Collection: Continue recording absorbance readings until the reaction nears completion or a sufficient number of data points are collected. Repeat this process at different temperatures (at least three different temperatures).
Data Analysis and Calculation
The collected data (time and absorbance) are used to determine the rate law and activation energy. This generally involves several steps:
1. Determining the Reaction Order:
- Method of Initial Rates: Analyze the initial rates of reaction at different initial concentrations of the reactants. Comparing the rates at varying concentrations allows the determination of reaction orders (m and n).
- Graphical Method (Integrated Rate Laws): For simple reactions (e.g., first-order or second-order), the integrated rate laws can be used. Plotting appropriate functions of concentration versus time (e.g., ln[A] vs. time for a first-order reaction) yields a straight line, and the slope provides information about the rate constant and reaction order.
2. Calculating the Rate Constant (k):
Once the reaction order is determined, the rate constant (k) can be calculated using the appropriate rate law equation and experimental data.
3. Determining the Activation Energy (Ea):
The Arrhenius equation is pivotal in determining the activation energy. Plotting ln(k) versus 1/T (where T is the absolute temperature) yields a straight line with a slope of -Ea/R. The slope of this line allows the calculation of Ea.
Sources of Error and Mitigation Strategies
Experimental errors can significantly impact the accuracy of the results. Common sources of error include:
- Inaccurate measurements: Errors in measuring reactant concentrations, temperature, and time can affect the calculated rate constant and activation energy. Using precise measuring instruments and techniques minimizes this error.
- Temperature fluctuations: Maintaining a constant temperature is crucial. Fluctuations can introduce error in determining the activation energy. Employing a thermostatically controlled water bath improves temperature stability.
- Incomplete mixing: Thoroughly mixing reactants is critical to ensure the reaction proceeds uniformly.
- Instrument errors: Calibration and proper functioning of the spectrophotometer are important. Regular calibration checks and instrument maintenance are recommended.
Advanced Considerations
Experiment 24 can be extended to explore more complex scenarios:
- Catalyst effects: Investigating the influence of catalysts on the reaction rate and activation energy.
- Reaction mechanism: Proposing a plausible reaction mechanism based on the determined rate law.
- Non-ideal behavior: Analyzing deviations from ideal behavior (e.g., due to ionic strength effects) and considering more sophisticated kinetic models.
Conclusion
Experiment 24 provides a valuable hands-on experience in understanding chemical kinetics. By meticulously following the procedure, analyzing the data effectively, and considering potential sources of error, students can gain a deeper understanding of rate laws, activation energy, and the factors influencing reaction rates. This knowledge is invaluable for various scientific and engineering applications. The ability to design, execute, and interpret such experiments is a critical skill for any aspiring scientist or engineer. Mastering this fundamental experiment lays a solid foundation for further exploration into the fascinating world of chemical kinetics and reaction dynamics.
Latest Posts
Latest Posts
-
Nervous System Anatomy And Physiology Quiz
May 23, 2025
-
To Apply For Brig Staff Duty
May 23, 2025
-
2 10 Unit Test Voices Of An Emerging Nation Part 1
May 23, 2025
-
Unit 2 The Living World Biodiversity Ap Exam Review
May 23, 2025
-
Which Of The Following Best Describes A Like Plan Change
May 23, 2025
Related Post
Thank you for visiting our website which covers about Experiment 24 A Rate Law And Activation Energy . We hope the information provided has been useful to you. Feel free to contact us if you have any questions or need further assistance. See you next time and don't miss to bookmark.