Exercise 13 Neuron Anatomy And Physiology
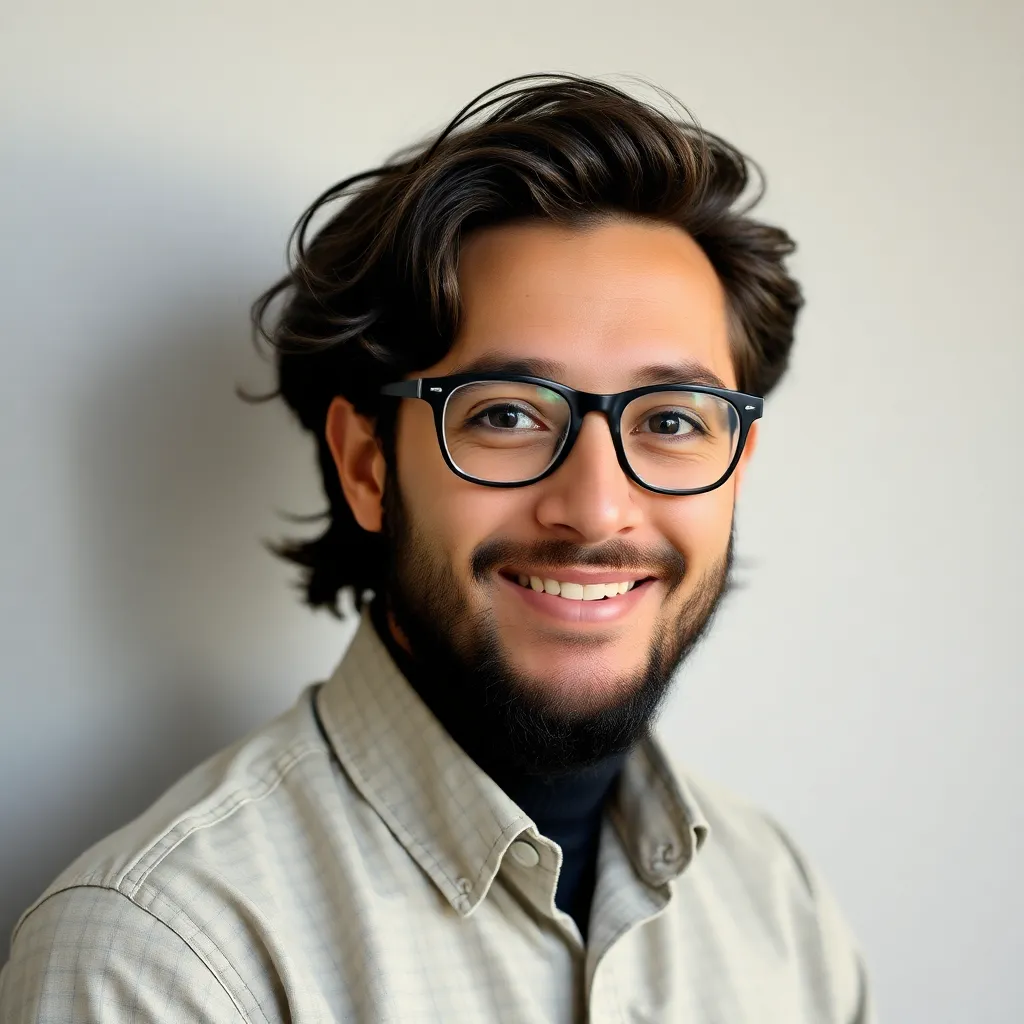
Juapaving
May 25, 2025 · 8 min read
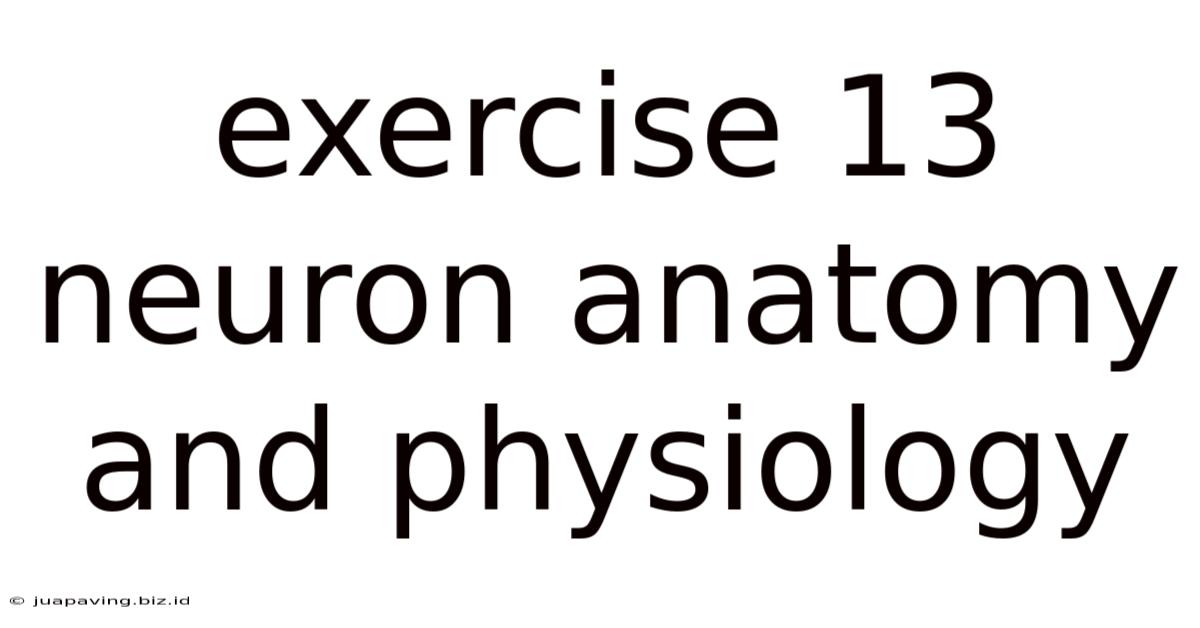
Table of Contents
Exercise 13: Delving into the Anatomy and Physiology of the Neuron
Understanding the neuron, the fundamental unit of the nervous system, is crucial for grasping the complexities of how our brains and bodies function. This comprehensive guide will delve into the intricate anatomy and physiology of neurons, providing a detailed exploration suitable for students and anyone fascinated by the intricacies of neuroscience. We'll cover everything from the structural components of a neuron to its electrical and chemical signaling processes.
I. Neuron Anatomy: The Building Blocks of the Nervous System
The neuron, a specialized cell, is responsible for receiving, processing, and transmitting information throughout the nervous system. Its unique structure is perfectly adapted to these functions. Let's examine its key components:
A. Soma (Cell Body): The Neuron's Control Center
The soma, or cell body, is the neuron's metabolic center. It contains the nucleus, which houses the genetic material (DNA), and various organelles crucial for cellular function, including:
- Nucleus: Contains the genetic blueprint for the neuron's structure and function.
- Mitochondria: Produce the energy (ATP) needed for neuronal activity.
- Ribosomes: Synthesize proteins essential for neuronal structure and function.
- Endoplasmic Reticulum: Involved in protein synthesis and transport.
- Golgi Apparatus: Processes and packages proteins for transport within and outside the neuron.
The soma integrates signals received from dendrites and initiates the transmission of signals down the axon. Its overall health and functioning are paramount to the neuron's ability to process and transmit information efficiently.
B. Dendrites: Receiving Information
Dendrites are branching extensions of the soma that receive signals from other neurons. Their extensive branching pattern significantly increases the surface area available for receiving synaptic inputs. The numerous dendritic spines further increase this surface area, allowing for a vast number of synaptic connections. These connections are crucial for integrating information from numerous other neurons. The shape and density of dendrites can vary significantly depending on the neuron's type and location in the nervous system, reflecting its specific functional role.
C. Axon: Transmitting Information
The axon is a long, slender projection that extends from the soma and transmits signals to other neurons, muscles, or glands. It's typically covered by a myelin sheath, a fatty insulating layer that significantly speeds up signal transmission. This myelin sheath is not continuous but rather segmented by nodes of Ranvier, gaps in the myelin where the axon membrane is exposed. These nodes play a critical role in the saltatory conduction of action potentials, which we'll discuss later.
D. Axon Terminal (Synaptic Terminals/Boutons): The Communication Hub
At the end of the axon, the axon branches into numerous axon terminals, also known as synaptic terminals or boutons. These terminals contain synaptic vesicles filled with neurotransmitters, chemical messengers that communicate with other neurons or target cells at the synapse. The synapse is the junction between the axon terminal of one neuron and the dendrite or soma of another neuron (or a muscle or gland cell). This is where information is transferred from one cell to another.
E. Myelin Sheath: Accelerating Signal Transmission
The myelin sheath, formed by glial cells (oligodendrocytes in the central nervous system and Schwann cells in the peripheral nervous system), acts as an insulating layer around the axon. This insulation dramatically increases the speed of signal transmission by allowing for saltatory conduction, where the action potential "jumps" from one node of Ranvier to the next. Damage to the myelin sheath, as seen in diseases like multiple sclerosis, can significantly impair neuronal signaling.
II. Neuron Physiology: The Electrical and Chemical Language of the Nervous System
The neuron's function depends on its ability to generate and transmit electrical and chemical signals. Let's delve into the fascinating processes involved:
A. Membrane Potential: The Electrical Gradient
The neuron's plasma membrane maintains an electrical potential difference, known as the membrane potential, between the inside and outside of the cell. This potential is primarily due to the unequal distribution of ions, particularly sodium (Na+), potassium (K+), chloride (Cl-), and calcium (Ca2+), across the membrane. The inside of the neuron is typically more negative than the outside, resulting in a resting membrane potential of approximately -70 mV. This negative resting potential is established and maintained by ion pumps and channels.
B. Ion Channels and Pumps: Regulating Ion Movement
The movement of ions across the neuronal membrane is tightly regulated by ion channels and pumps:
- Ion Channels: These protein pores selectively allow specific ions to pass through the membrane. Some channels are always open (leak channels), while others are gated, opening or closing in response to specific stimuli (voltage, ligand, or mechanical).
- Ion Pumps: These protein complexes actively transport ions against their concentration gradients, requiring energy (ATP). The sodium-potassium pump (Na+/K+ pump) is a crucial example, maintaining the resting membrane potential by pumping three Na+ ions out and two K+ ions into the cell.
C. Action Potential: The Nerve Impulse
An action potential is a rapid, transient reversal of the membrane potential that propagates along the axon. It's the fundamental signal used for long-distance communication in the nervous system. The action potential is triggered when the membrane potential reaches a threshold level, typically around -55 mV. This threshold triggers a cascade of events involving the opening and closing of voltage-gated ion channels:
- Depolarization: Voltage-gated Na+ channels open, allowing a massive influx of Na+ ions into the cell, rapidly depolarizing the membrane potential to a positive value (+30 mV).
- Repolarization: Voltage-gated Na+ channels inactivate, and voltage-gated K+ channels open, allowing a rapid outflow of K+ ions, repolarizing the membrane potential back towards its resting value.
- Hyperpolarization: The outflow of K+ ions can briefly hyperpolarize the membrane potential below the resting level before it gradually returns to its resting state.
This sequence of events constitutes a single action potential. The action potential is an "all-or-none" event; it either occurs fully or not at all. Its amplitude and duration are relatively constant, encoding information in the frequency of action potentials rather than their amplitude. The speed of action potential propagation is increased by the myelin sheath, which allows for saltatory conduction.
D. Synaptic Transmission: Chemical Communication at the Synapse
Once the action potential reaches the axon terminal, it triggers the release of neurotransmitters into the synaptic cleft, the space between the pre-synaptic neuron (the one sending the signal) and the post-synaptic neuron (the one receiving the signal). This process is called synaptic transmission and involves several steps:
- Action Potential Arrival: The action potential depolarizes the axon terminal.
- Calcium Influx: Voltage-gated Ca2+ channels open, allowing Ca2+ ions to enter the axon terminal.
- Vesicle Fusion: The influx of Ca2+ triggers the fusion of synaptic vesicles with the pre-synaptic membrane, releasing neurotransmitters into the synaptic cleft.
- Neurotransmitter Binding: Neurotransmitters diffuse across the synaptic cleft and bind to receptors on the post-synaptic membrane.
- Postsynaptic Potential: Neurotransmitter binding can lead to either excitatory postsynaptic potentials (EPSPs), depolarizing the postsynaptic membrane, or inhibitory postsynaptic potentials (IPSPs), hyperpolarizing the postsynaptic membrane.
- Neurotransmitter Removal: Neurotransmitters are removed from the synaptic cleft through reuptake, enzymatic degradation, or diffusion.
The summation of EPSPs and IPSPs determines whether the postsynaptic neuron will fire an action potential. If the combined effect of EPSPs is sufficient to reach the threshold potential, the postsynaptic neuron will fire an action potential, propagating the signal.
III. Types of Neurons and Their Functions
Neurons are not all alike. They exhibit diverse morphologies and functional specializations, reflecting their roles in the nervous system. Some common types include:
- Sensory Neurons: Transmit information from sensory receptors to the central nervous system. They are responsible for our senses of sight, hearing, touch, taste, and smell.
- Motor Neurons: Transmit signals from the central nervous system to muscles and glands, controlling movement and physiological responses.
- Interneurons: Connect sensory and motor neurons, processing information within the central nervous system. They are responsible for complex integration and processing of information.
The diversity in neuron structure and function underlines the intricate complexity of the nervous system, allowing for a wide range of behaviors and functions. Understanding these diverse types and their interactions is key to understanding the brain's overall function.
IV. Clinical Significance of Neuron Dysfunction
Proper neuron function is paramount for maintaining overall health. Dysfunction in neuronal activity can lead to a variety of neurological disorders, including:
- Multiple Sclerosis (MS): An autoimmune disease that damages the myelin sheath, leading to impaired signal transmission and neurological symptoms.
- Alzheimer's Disease: A neurodegenerative disease characterized by the accumulation of amyloid plaques and neurofibrillary tangles, leading to neuronal loss and cognitive decline.
- Parkinson's Disease: A neurodegenerative disease caused by the loss of dopamine-producing neurons in the substantia nigra, leading to motor impairments.
- Epilepsy: A neurological disorder characterized by recurrent seizures, which are abnormal electrical discharges in the brain.
These examples highlight the critical role neurons play in our health and well-being. Research into neuronal function and dysfunction is crucial for developing effective treatments and cures for neurological disorders.
V. Conclusion: The Intricate World of the Neuron
This in-depth exploration of neuron anatomy and physiology reveals the remarkable complexity and sophistication of these fundamental units of the nervous system. From their intricate structure to their precise mechanisms of electrical and chemical signaling, neurons are truly fascinating cells. Their proper functioning is essential for all aspects of our physical and cognitive abilities. Further research into their intricacies promises to unlock even greater understanding of the human brain and nervous system, ultimately paving the way for advancements in treating neurological disorders and improving human health. This exercise provides a solid foundation for continued exploration into this captivating field.
Latest Posts
Latest Posts
-
Single Parents Who Cannot Deploy May Be Discharged
May 25, 2025
-
Which Of The Following Is True Of Defense Mechanisms
May 25, 2025
-
Handsomest Drowned Man In The World Analysis
May 25, 2025
-
Which Of The Following Is Not True Of Graded Potentials
May 25, 2025
-
What Does The Scaffold Symbolize In The Scarlet Letter
May 25, 2025
Related Post
Thank you for visiting our website which covers about Exercise 13 Neuron Anatomy And Physiology . We hope the information provided has been useful to you. Feel free to contact us if you have any questions or need further assistance. See you next time and don't miss to bookmark.