Energy Band Diagram Of A Semiconductor
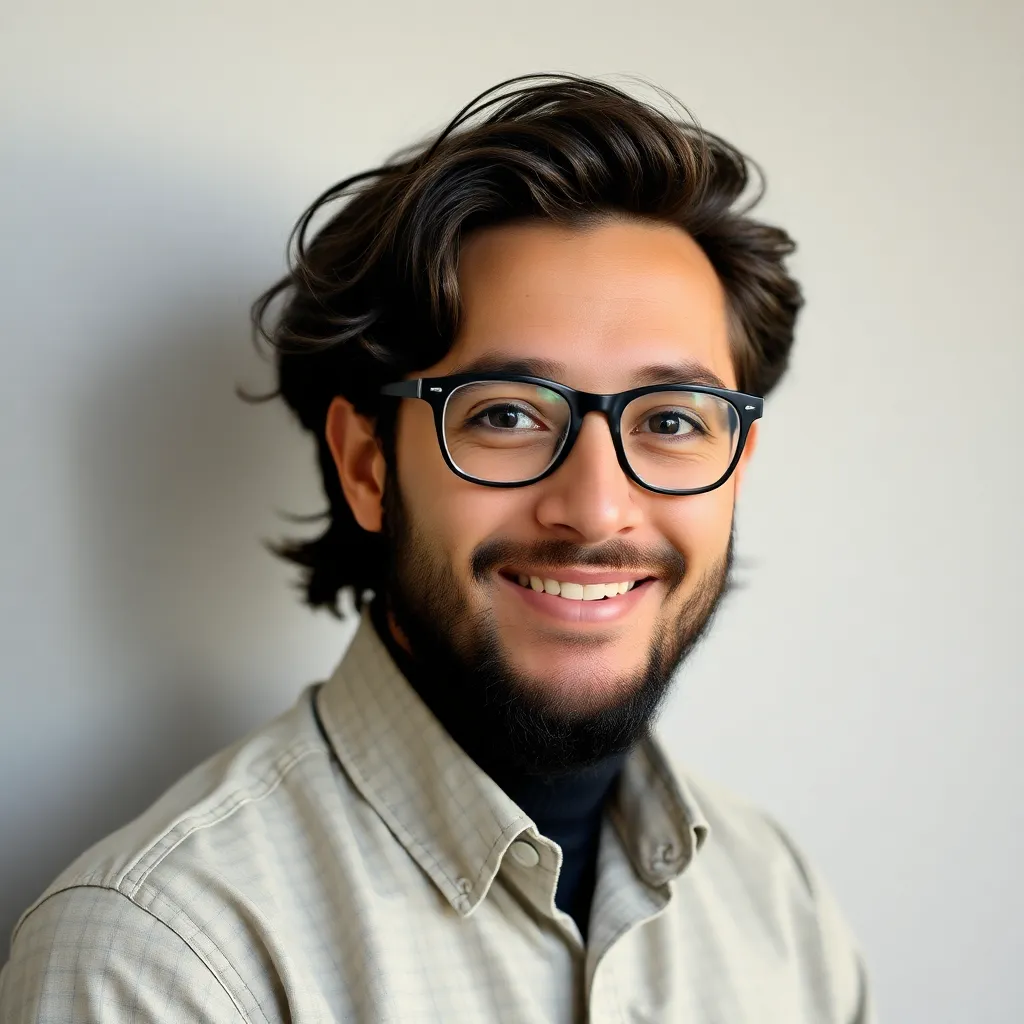
Juapaving
May 13, 2025 · 6 min read

Table of Contents
Energy Band Diagram of a Semiconductor: A Deep Dive
The energy band diagram is a crucial tool for understanding the electrical and optical properties of semiconductors. It provides a visual representation of the allowed energy levels for electrons within a material, revealing key information about its conductivity, band gap, and potential applications in various electronic and optoelectronic devices. This comprehensive guide will explore the energy band diagram in detail, covering its construction, interpretation, and significance in different semiconductor types.
Understanding the Fundamentals
Before delving into the intricacies of energy band diagrams, let's establish a solid foundation. The behavior of electrons in a solid is governed by quantum mechanics. In an isolated atom, electrons occupy discrete energy levels. However, when numerous atoms come together to form a solid, these discrete levels broaden into energy bands due to interactions between neighboring atoms.
Valence Band and Conduction Band
The energy band diagram primarily features two key bands:
-
Valence Band: This band represents the range of energy levels occupied by the valence electrons – the electrons involved in chemical bonding within the solid. A completely filled valence band signifies an insulator or a semiconductor at absolute zero temperature.
-
Conduction Band: This band lies above the valence band and represents the energy levels available for electrons to freely move and conduct electricity. Electrons in the conduction band are not bound to specific atoms and contribute to the material's conductivity.
Band Gap (Eg)
The crucial difference between insulators, semiconductors, and conductors lies in the energy gap, or band gap (Eg), separating the valence and conduction bands.
-
Insulators: Insulators have a large band gap (typically > 4 eV). At room temperature, very few electrons possess sufficient energy to jump across this gap from the valence to the conduction band, resulting in extremely low conductivity.
-
Semiconductors: Semiconductors possess a smaller band gap (typically 0.1 eV to 4 eV). At room temperature, a significant number of electrons can gain enough thermal energy to overcome this gap, leading to moderate conductivity. This conductivity can be further enhanced by doping.
-
Conductors: Conductors have overlapping valence and conduction bands, meaning no energy gap exists. Electrons can easily move between these bands, resulting in high conductivity.
Constructing the Energy Band Diagram
The energy band diagram is a simplified representation, but its construction requires careful consideration of various factors. The diagram typically plots energy (E) on the vertical axis and position (x) on the horizontal axis, illustrating how energy levels vary across different regions of the material.
Intrinsic Semiconductors
An intrinsic semiconductor is a pure semiconductor without any intentional doping. Its energy band diagram at absolute zero temperature shows a completely filled valence band and an empty conduction band, with the band gap clearly separating them. At higher temperatures, thermal excitation promotes some electrons from the valence band to the conduction band, creating electron-hole pairs.
Extrinsic Semiconductors
Extrinsic semiconductors are created by intentionally introducing impurities, or dopants, into an intrinsic semiconductor to alter its electrical properties. There are two primary types of doping:
-
n-type doping: Introducing donor impurities (e.g., phosphorus in silicon) with extra valence electrons increases the number of electrons in the conduction band, creating a majority of electrons and a minority of holes. The energy band diagram shows a donor energy level just below the conduction band.
-
p-type doping: Introducing acceptor impurities (e.g., boron in silicon) with fewer valence electrons creates "holes" – empty energy levels – in the valence band. These holes act as mobile positive charges. The energy band diagram shows an acceptor energy level just above the valence band.
pn-junction
The pn-junction is formed by joining p-type and n-type semiconductors. This interface creates a crucial depletion region where the majority carriers diffuse across the junction, leaving behind immobile ionized impurities. The energy band diagram of a pn-junction shows a bending of the bands at the junction due to the built-in potential, which arises from the diffusion of charge carriers. This built-in potential is responsible for the rectifying behavior of the pn-junction.
Interpreting the Energy Band Diagram
The energy band diagram is not merely a visual representation; it's a powerful tool for understanding various semiconductor phenomena:
-
Carrier Concentration: The difference between the Fermi level (the energy level at which the probability of an electron occupying a state is 50%) and the band edges provides information about the carrier concentration in the conduction and valence bands.
-
Band Bending: Band bending at junctions (like pn-junctions) indicates the presence of electric fields and potential barriers. The magnitude of band bending reveals information about the strength of the electric field.
-
Carrier Transport: The slopes of the bands represent the electric field, influencing how charge carriers move across different regions of the material.
-
Optical Transitions: The band gap dictates the energy required for electrons to transition from the valence band to the conduction band, directly influencing the material's optical properties, such as absorption and emission of light.
Applications of Energy Band Diagrams
Energy band diagrams are essential in understanding and designing numerous semiconductor devices:
-
Diodes: The rectifying behavior of diodes is directly related to the band bending at the pn-junction.
-
Transistors: Understanding the energy band diagrams of transistors is crucial in analyzing their current amplification capabilities.
-
Solar Cells: The band gap of the semiconductor material in a solar cell determines the range of wavelengths of light that can be effectively absorbed, influencing the cell's efficiency.
-
LEDs (Light Emitting Diodes): The band gap of the semiconductor material directly determines the color of the light emitted by an LED.
-
Photodetectors: The band gap is crucial in determining which wavelengths of light a photodetector can effectively detect.
Advanced Concepts
While the basic principles outlined above provide a solid foundation, more advanced concepts further refine our understanding of energy band diagrams:
-
Effective Mass: Electrons and holes don't behave exactly like free particles; their effective mass reflects how they respond to external forces within the crystal lattice. The effective mass can affect the shape of the energy bands.
-
Density of States: The density of states indicates the number of available energy levels per unit volume at a particular energy. It plays a significant role in determining carrier statistics.
-
k-space Diagrams: These more advanced diagrams consider the momentum (k) of electrons alongside energy, providing a more detailed description of the band structure.
-
Heterojunctions: These involve junctions between two different semiconductor materials, creating more complex band diagrams and leading to enhanced device capabilities.
Conclusion
The energy band diagram is an indispensable tool for understanding and engineering semiconductor devices. Its seemingly simple representation encapsulates a wealth of information regarding electron behavior, charge transport, optical properties, and ultimately, device performance. By mastering the interpretation of these diagrams, scientists and engineers can design and optimize a wide range of electronic and optoelectronic technologies. From the fundamental principles of intrinsic and extrinsic semiconductors to the complexities of pn-junctions and advanced device applications, a thorough grasp of the energy band diagram is essential for anyone working in the field of semiconductor physics and engineering. Continuous advancements in semiconductor technology rely on the ability to manipulate and understand the intricacies of these diagrams, pushing the boundaries of innovation in electronics and photonics.
Latest Posts
Latest Posts
-
Which Of The Following Statements Is True About
May 13, 2025
-
Why Is The Blood Testis Barrier Important
May 13, 2025
-
What Is The Strongest Type Of Intermolecular Force
May 13, 2025
-
Animals That Cannot Make Their Own Food Are Called
May 13, 2025
-
How Many Obtuse Angles Are In A Obtuse Triangle
May 13, 2025
Related Post
Thank you for visiting our website which covers about Energy Band Diagram Of A Semiconductor . We hope the information provided has been useful to you. Feel free to contact us if you have any questions or need further assistance. See you next time and don't miss to bookmark.