Electron Configuration For Copper And Chromium
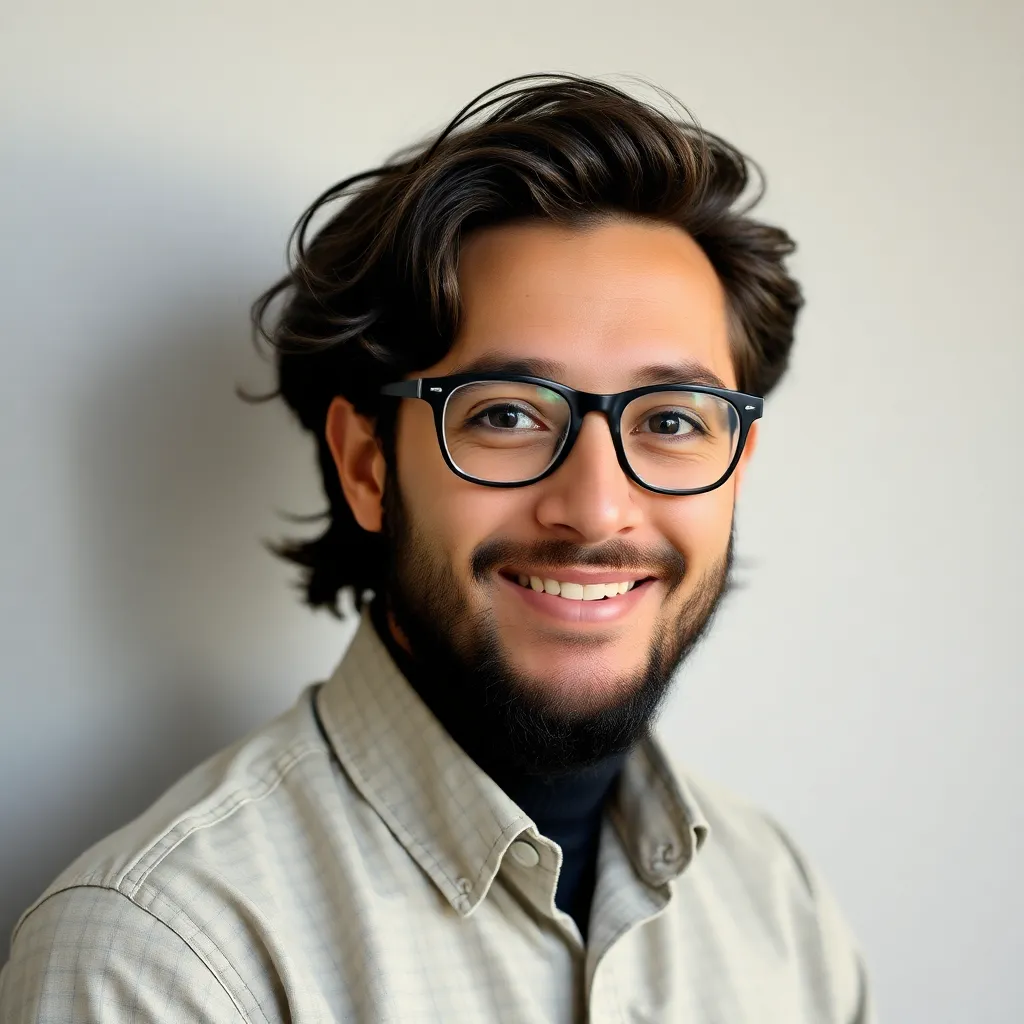
Juapaving
Apr 27, 2025 · 6 min read

Table of Contents
Electron Configuration: The Curious Cases of Copper and Chromium
The periodic table, a seemingly simple arrangement of elements, hides a world of complex electron behavior. While the Aufbau principle and Hund's rule generally predict electron configurations accurately, certain elements, particularly chromium (Cr) and copper (Cu), defy these rules, showcasing exceptions that deepen our understanding of atomic structure and stability. This article delves into the electron configurations of copper and chromium, exploring the reasons behind their unusual arrangements and the implications for their chemical properties.
Understanding Electron Configuration Basics
Before diving into the exceptions, let's establish a foundational understanding of electron configuration. Electron configuration describes the arrangement of electrons in an atom's energy levels and sublevels. Electrons occupy orbitals within these sublevels, following specific rules:
- Aufbau Principle: Electrons fill orbitals starting with the lowest energy level and progressing upwards.
- Pauli Exclusion Principle: Each orbital can hold a maximum of two electrons with opposite spins.
- Hund's Rule: Within a sublevel, electrons occupy orbitals individually before pairing up.
These rules, when applied, generally predict the electron configuration accurately. For example, the predicted electron configuration for a neutral atom of iron (Fe, atomic number 26) is 1s²2s²2p⁶3s²3p⁶4s²3d⁶.
The Anomaly of Chromium (Cr, Atomic Number 24)
Chromium, with 24 electrons, presents the first significant deviation from the standard Aufbau principle. The predicted configuration, based on filling orbitals in order of increasing energy, would be [Ar] 4s²3d⁴. However, the observed, experimentally verified configuration is [Ar] 3d⁵4s¹.
Why this exception? The answer lies in the subtle energy differences between the 3d and 4s orbitals. While the 4s orbital generally has lower energy than the 3d, this difference is small. In chromium, the additional stability gained by having a half-filled 3d subshell (five electrons, each in a separate orbital) outweighs the slight energy advantage of filling the 4s orbital completely. A half-filled or completely filled subshell leads to enhanced stability due to increased electron-electron repulsion and exchange energy. This enhanced stability is a significant factor in explaining the observed electron configuration.
Implications of Chromium's Electron Configuration
This unusual configuration influences chromium's chemical behavior. The availability of a single 4s electron contributes to chromium's ability to form various oxidation states, including +2, +3, and +6. This versatility in oxidation states contributes to chromium's diverse applications in various industries. For example, the +3 oxidation state is common in various chromium compounds, whilst the +6 oxidation state is important in chromates and dichromates. This versatility is directly linked to its unique electron configuration.
The Case of Copper (Cu, Atomic Number 29)
Similar to chromium, copper exhibits an unexpected electron configuration. The predicted configuration would be [Ar] 4s²3d⁹. However, the experimentally observed configuration is [Ar] 3d¹⁰4s¹.
The reason behind this anomaly is parallel to that of chromium. The energy difference between the 4s and 3d orbitals is small. In copper's case, the exceptional stability of a completely filled 3d subshell (ten electrons) surpasses the slight energy advantage of a filled 4s orbital. The extra stability provided by a full d-subshell trumps the conventional Aufbau order.
Consequences of Copper's Electron Configuration
Like chromium, copper's unusual electron configuration has significant implications for its chemical properties. The presence of a single electron in the 4s orbital allows for a +1 oxidation state, while the filled 3d shell contributes to its stability in its +2 oxidation state. This contributes to the metal's excellent conductivity and its widespread use in electrical wiring. The filled d-orbital also contributes to its distinctive reddish-pink color. The stability of the full d-subshell also makes it relatively unreactive compared to other transition metals.
Comparing Chromium and Copper
Both chromium and copper display an exception to the Aufbau principle, illustrating the complexities of electron behavior. While the underlying principle driving the exceptions is the same – the exceptional stability of half-filled and completely filled subshells – the consequences are unique to each element.
Feature | Chromium (Cr) | Copper (Cu) |
---|---|---|
Predicted Configuration | [Ar] 4s²3d⁴ | [Ar] 4s²3d⁹ |
Observed Configuration | [Ar] 3d⁵4s¹ | [Ar] 3d¹⁰4s¹ |
Reason for Exception | Stability of half-filled 3d | Stability of completely filled 3d |
Common Oxidation States | +2, +3, +6 | +1, +2 |
Chemical Implications | Diverse oxidation states, varied applications | Excellent conductivity, stability |
Beyond the Exceptions: A Broader Perspective
The unusual electron configurations of chromium and copper highlight the limitations of simplistic rules like the Aufbau principle. While these rules offer a valuable framework for understanding electron arrangements, they are not absolute. The interplay between electron-electron interactions and orbital energy differences plays a crucial role in determining the actual electronic structure of atoms. This underscores the dynamic and intricate nature of atomic behavior.
The deviations observed in chromium and copper aren't isolated cases; other transition metals also exhibit similar irregularities. The complexities of electron-electron repulsion, exchange energy, and subtle orbital energy differences make precise predictions challenging. Advanced computational methods and spectroscopic techniques are often employed to determine accurate electron configurations.
Implications for Chemical Reactivity and Bonding
The unusual electron configurations of chromium and copper have profound implications for their chemical reactivity and bonding behavior. The availability of electrons in the outermost shell, influenced by the configuration, dictates the nature and number of bonds these elements can form. The exceptional stability of a half-filled or completely filled d-subshell also affects the strength and stability of these bonds.
For instance, the multiple oxidation states exhibited by chromium, a direct consequence of its electronic structure, enable it to participate in a wide array of chemical reactions and form diverse compounds with varying properties. Similarly, copper's unique configuration contributes to its excellent electrical and thermal conductivity, making it indispensable in various applications.
Conclusion: A Deeper Dive into Atomic Behavior
The electron configurations of chromium and copper serve as compelling examples of how the seemingly straightforward rules governing electron arrangements can be nuanced and complex. These exceptions highlight the need for a deeper understanding of atomic structure, going beyond simple mnemonic devices to embrace the intricacies of electron-electron interactions and orbital energy levels. Understanding these subtleties is essential for predicting and explaining the chemical and physical properties of these elements and their wider roles in materials science and industrial applications. By studying these exceptions, we develop a richer and more accurate picture of the intricate world of atomic physics and chemistry. The journey of understanding the nuances of electron configurations continues, pushing the boundaries of our knowledge and innovation.
Latest Posts
Latest Posts
-
How Many Radians Is 30 Degrees
Apr 28, 2025
-
Talk Out Of 7 Little Words
Apr 28, 2025
-
The Correct Order Of Events In Aerobic Respiration Is
Apr 28, 2025
-
2 5 Kg Is How Many Grams
Apr 28, 2025
-
Is Photosynthesis An Exothermic Or Endothermic Reaction
Apr 28, 2025
Related Post
Thank you for visiting our website which covers about Electron Configuration For Copper And Chromium . We hope the information provided has been useful to you. Feel free to contact us if you have any questions or need further assistance. See you next time and don't miss to bookmark.