Dna Is A Polymer Made Of Monomers Called
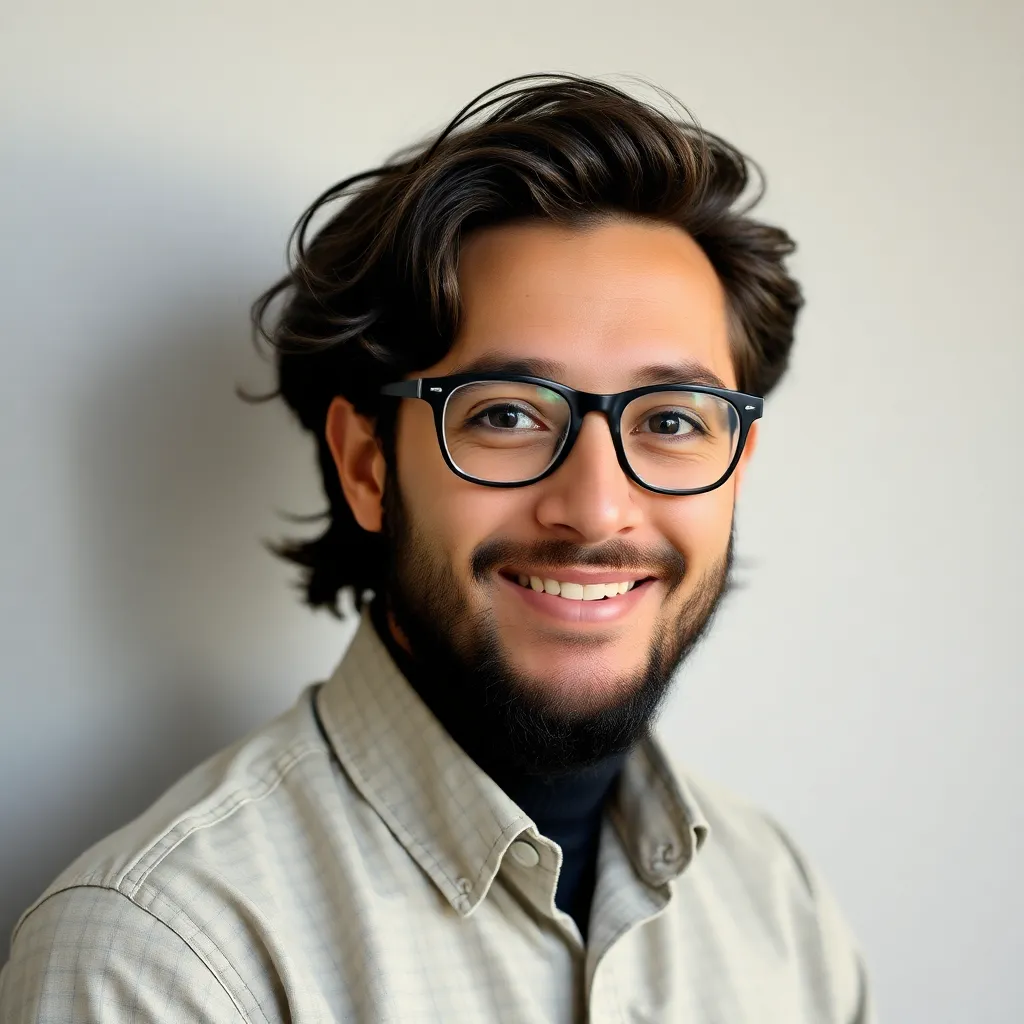
Juapaving
May 09, 2025 · 7 min read
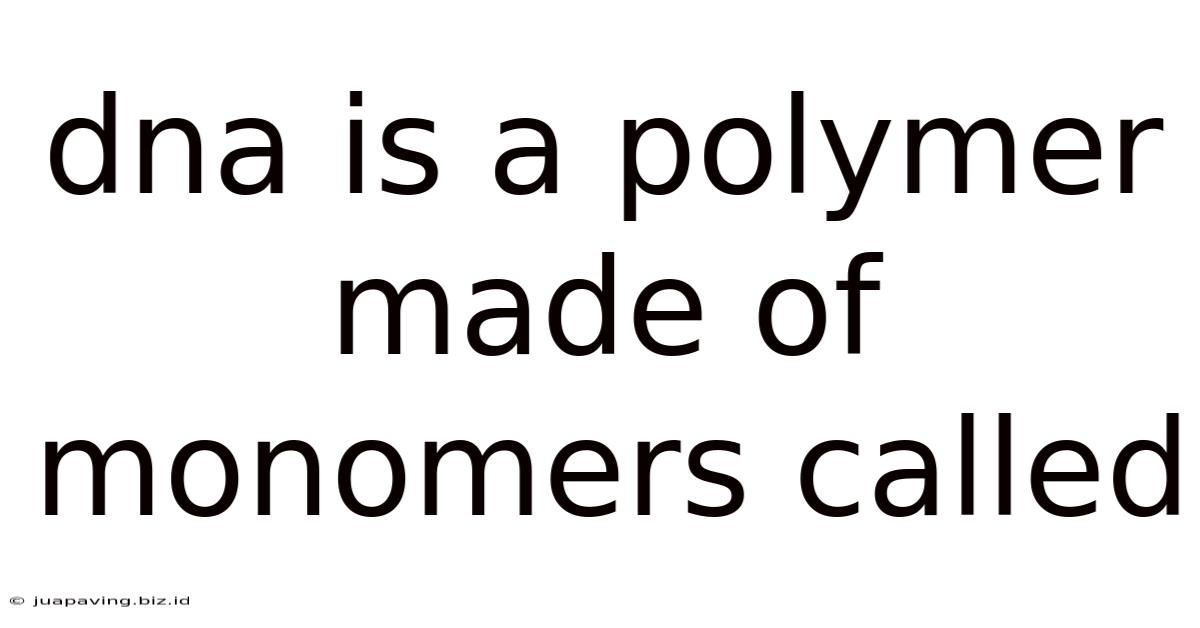
Table of Contents
DNA: A Polymer Made of Monomers Called Nucleotides
Deoxyribonucleic acid, or DNA, is the fundamental building block of life. It's a molecule that carries the genetic instructions for the development, functioning, growth, and reproduction of all known organisms and many viruses. But what exactly is DNA? At its core, DNA is a polymer, a large molecule composed of repeating structural units. These units are called monomers, and in the case of DNA, these monomers are nucleotides.
Understanding Polymers and Monomers
Before delving into the specifics of DNA nucleotides, let's establish a basic understanding of polymers and monomers. A polymer is a large molecule, or macromolecule, composed of many smaller, repeating subunits called monomers. Think of it like a long train, where each carriage represents a monomer, and the entire train is the polymer. Many biological molecules are polymers, including proteins (made of amino acid monomers), carbohydrates (made of sugar monomers), and nucleic acids (made of nucleotide monomers). DNA and RNA are both types of nucleic acids.
The Structure of a Nucleotide: The Building Block of DNA
A nucleotide is the monomeric unit of DNA. Each nucleotide consists of three components:
1. A Pentose Sugar: Deoxyribose
The sugar component in DNA is deoxyribose, a five-carbon sugar (pentose). The term "deoxyribose" indicates that it's a modified version of ribose, the sugar found in RNA. The difference lies in the presence of a hydroxyl (-OH) group on the 2' carbon in ribose, which is replaced by a hydrogen atom (-H) in deoxyribose. This seemingly small difference has significant implications for the stability and function of DNA. Deoxyribose's structure contributes to the overall stability of the DNA double helix.
2. A Phosphate Group
The phosphate group is a negatively charged molecule (PO₄³⁻). It's crucial for the backbone structure of the DNA polymer. The phosphate groups link the sugar molecules of adjacent nucleotides, forming a sugar-phosphate backbone that runs along the length of the DNA molecule. This backbone provides structural support and also plays a role in the interaction of DNA with proteins. The negative charge of the phosphate groups contributes to the overall negative charge of the DNA molecule, affecting its behavior in aqueous solutions.
3. A Nitrogenous Base: Adenine, Guanine, Cytosine, or Thymine
This is perhaps the most important component for carrying genetic information. The nitrogenous base is a nitrogen-containing organic molecule that comes in four varieties in DNA:
- Adenine (A): A purine base, characterized by a double-ring structure.
- Guanine (G): Another purine base, also with a double-ring structure.
- Cytosine (C): A pyrimidine base, with a single-ring structure.
- Thymine (T): A pyrimidine base, also with a single-ring structure.
These bases are crucial because they form specific pairs with each other through hydrogen bonds, a key aspect of DNA's double helix structure.
The DNA Double Helix: From Monomers to a Functional Molecule
The nucleotides don't exist in isolation; they are joined together to form long chains. The phosphate group of one nucleotide forms a covalent bond with the 3' carbon of the sugar in the next nucleotide, creating a phosphodiester bond. This linkage results in a directional chain with a 5' end (where the phosphate group is attached to the 5' carbon of the sugar) and a 3' end (where the sugar's 3' carbon is free).
Two of these polynucleotide chains then intertwine to form the iconic double helix structure of DNA. This structure is stabilized by hydrogen bonds between the nitrogenous bases of the two strands. Adenine (A) always pairs with thymine (T) via two hydrogen bonds, while guanine (G) always pairs with cytosine (C) via three hydrogen bonds. This specific base pairing, known as Chargaff's rules, is fundamental to DNA replication and gene expression. The double helix structure provides a compact and efficient way to store and protect the genetic information.
The Significance of Nucleotide Sequence: The Language of Life
The specific order, or sequence, of nucleotides along the DNA strand determines the genetic information. This sequence dictates the order of amino acids in proteins, which in turn determine the protein's function. The sequence also controls other aspects of cellular processes, such as gene regulation and the timing of developmental events. Changes in the nucleotide sequence, known as mutations, can have profound effects on an organism, ranging from minor changes in phenotype to lethal consequences. The precise arrangement of these nucleotides forms the basis of the genetic code, a system that translates the nucleotide sequence into the amino acid sequence of proteins.
DNA Replication: Passing on the Genetic Blueprint
The double-helical structure of DNA is perfectly suited for replication, the process by which a cell makes an identical copy of its DNA before cell division. During replication, the two DNA strands separate, and each strand serves as a template for the synthesis of a new complementary strand. Enzymes called DNA polymerases catalyze the addition of new nucleotides to the growing strand, following the base-pairing rules (A with T, and G with C). This results in two identical DNA molecules, each consisting of one original strand and one newly synthesized strand – a process known as semi-conservative replication. The fidelity of this process is crucial for maintaining the integrity of the genetic information across generations.
DNA and Gene Expression: From Blueprint to Functional Product
The information encoded in the DNA sequence is not directly used to build proteins. Instead, it's first transcribed into a messenger RNA (mRNA) molecule, a process carried out by an enzyme called RNA polymerase. mRNA is a single-stranded nucleic acid similar to DNA but contains uracil (U) instead of thymine (T). The mRNA molecule then carries the genetic information from the DNA to the ribosomes, the protein synthesis machinery of the cell.
At the ribosome, the mRNA sequence is translated into a protein. This process involves transfer RNA (tRNA) molecules, which bring specific amino acids to the ribosome according to the mRNA sequence. Each three-nucleotide sequence on the mRNA, called a codon, specifies a particular amino acid. The ribosome links these amino acids together to form a polypeptide chain, which folds into a functional protein. This intricate process of transcription and translation transforms the genetic information encoded in DNA's nucleotide sequence into the functional proteins that carry out the cell's activities.
Beyond the Basics: Variations and Modifications
While the basic structure of DNA—a polymer of nucleotides composed of deoxyribose sugar, a phosphate group, and a nitrogenous base—is conserved across all life forms, variations and modifications exist.
-
Epigenetics: Chemical modifications to DNA or associated histone proteins can alter gene expression without changing the underlying DNA sequence. These modifications include methylation of cytosine bases and various histone modifications, affecting how tightly DNA is packaged and influencing the accessibility of genes to transcription machinery. These epigenetic changes play a crucial role in development, disease, and environmental responses.
-
Alternative splicing: A single gene can encode multiple protein isoforms through alternative splicing of mRNA. This process involves selectively including or excluding certain exons (coding regions) during mRNA processing, resulting in different mRNA molecules and, consequently, different proteins.
-
Non-coding DNA: A significant portion of the genome does not code for proteins but plays essential regulatory roles, including gene regulation, chromosome structure, and other cellular processes. These non-coding regions contain various elements like promoters, enhancers, silencers, and other regulatory sequences that influence gene expression.
DNA's Role in Various Fields
The understanding of DNA's structure and function has revolutionized numerous fields:
-
Medicine: DNA sequencing and analysis are crucial for diagnosing genetic disorders, identifying disease susceptibility, and developing personalized medicine approaches. Gene therapy aims to correct genetic defects by introducing functional genes into cells.
-
Forensics: DNA fingerprinting is a powerful tool used in criminal investigations and paternity testing. DNA analysis provides a highly accurate way to identify individuals based on their unique genetic profiles.
-
Agriculture: Genetic engineering techniques modify crops to enhance yield, improve nutritional content, and increase resistance to pests and diseases.
-
Evolutionary Biology: Comparative analysis of DNA sequences reveals evolutionary relationships between species and helps reconstruct evolutionary history.
Conclusion
DNA, a polymer composed of monomers called nucleotides, is the cornerstone of life. Its elegant double-helix structure, the specific base pairing rules, and the intricate mechanisms of replication, transcription, and translation, enable the storage, replication, and expression of genetic information. Understanding DNA's structure and function is fundamental to comprehending biology and has profound implications across various scientific disciplines. Further research continues to unveil the complexities and subtleties of this remarkable molecule, promising further advances in our understanding of life itself. The ongoing exploration of DNA's intricacies reveals its astonishing power and its crucial role in shaping life as we know it. From its fundamental structure to its profound implications for numerous fields, DNA remains a captivating subject of ongoing study and discovery.
Latest Posts
Latest Posts
-
How To Find The Complementary Dna Strand
May 10, 2025
-
The Main Products Of Photosynthesis Are
May 10, 2025
-
5 1 2 As A Percent
May 10, 2025
-
Why Do Some Cells Have More Mitochondria Give An Example
May 10, 2025
-
How Many Mm Is 12 Cm
May 10, 2025
Related Post
Thank you for visiting our website which covers about Dna Is A Polymer Made Of Monomers Called . We hope the information provided has been useful to you. Feel free to contact us if you have any questions or need further assistance. See you next time and don't miss to bookmark.