Diffusion Of Respiratory Gases Takes Place At The
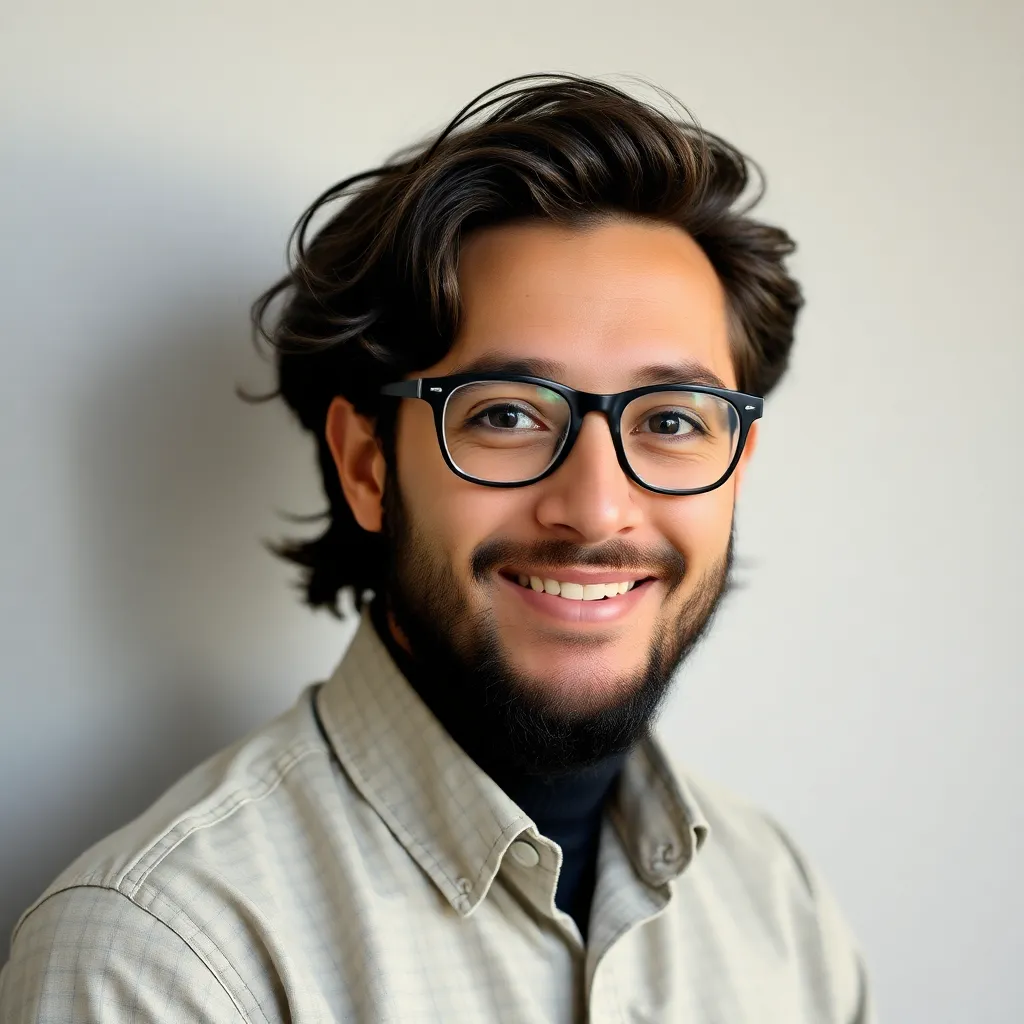
Juapaving
Apr 17, 2025 · 7 min read

Table of Contents
Diffusion of Respiratory Gases Takes Place at the Alveoli: A Deep Dive into Pulmonary Gas Exchange
The miracle of breathing, seemingly effortless, is a complex orchestration of physiological processes. At the heart of this process lies the exchange of respiratory gases – oxygen (O2) and carbon dioxide (CO2) – between the air we breathe and our bloodstream. This crucial event, responsible for sustaining life, occurs primarily at the alveoli, tiny air sacs within our lungs. This article will delve deep into the process of diffusion of respiratory gases at the alveoli, exploring the underlying mechanisms, influencing factors, and clinical implications.
Understanding the Alveoli: The Site of Gas Exchange
The lungs, far from being simple balloon-like structures, are intricate organs composed of millions of alveoli. These tiny, balloon-shaped structures are clustered together like grapes on a vine, providing an enormous surface area for gas exchange. This vast surface area – approximately 70 square meters in adults – is critical because diffusion relies on the availability of a large contact area between the air and blood.
Alveolar Structure and Function
Each alveolus is surrounded by a network of capillaries, the smallest blood vessels in the body. The walls of both alveoli and capillaries are exceptionally thin, consisting of a single layer of epithelial cells, known as the alveolar epithelium and the capillary endothelium, respectively. This thinness, along with the close proximity of the air and blood, minimizes the distance that gases need to travel during diffusion, making the process highly efficient. The alveolar epithelium also contains specialized cells called type I pneumocytes which form the majority of the alveolar surface area, optimizing gas exchange. Type II pneumocytes, on the other hand, are responsible for producing surfactant, a crucial substance that reduces surface tension within the alveoli, preventing their collapse during exhalation.
The Respiratory Membrane: A Bridge Between Air and Blood
The combined structure of the alveolar epithelium, the capillary endothelium, and their intervening basement membranes constitutes the respiratory membrane, also known as the alveolocapillary membrane. This extremely thin membrane (approximately 0.5 µm) is the critical interface where gas exchange occurs. The thinness of the respiratory membrane is vital for efficient diffusion, as the rate of diffusion is inversely proportional to the distance gases must travel. Any thickening of this membrane, such as in certain lung diseases, can significantly impair gas exchange.
The Physics of Diffusion: Driving Gas Exchange
The movement of respiratory gases across the alveolocapillary membrane is governed by the principles of diffusion, a passive process driven by differences in partial pressures. Partial pressure is the pressure exerted by an individual gas within a mixture of gases. In the context of respiration, the partial pressures of oxygen (PO2) and carbon dioxide (PCO2) are crucial determinants of gas exchange.
Partial Pressure Gradients: The Driving Force
Air entering the alveoli has a higher PO2 (approximately 100 mmHg) than the deoxygenated blood arriving in the pulmonary capillaries (approximately 40 mmHg). This difference in partial pressure creates a pressure gradient, driving oxygen to diffuse from the alveoli into the blood. Conversely, the PCO2 in the arriving blood (approximately 46 mmHg) is higher than that in the alveolar air (approximately 40 mmHg), resulting in a pressure gradient that promotes the diffusion of CO2 from the blood into the alveoli. These pressure gradients are the fundamental driving force behind respiratory gas exchange.
Factors Affecting Diffusion Rate
Several factors influence the rate of diffusion across the respiratory membrane:
-
Surface Area: As mentioned previously, the vast surface area of the alveoli is crucial for efficient gas exchange. Any reduction in this surface area, such as in emphysema (a condition where alveolar walls are destroyed), significantly impairs diffusion.
-
Thickness of the Respiratory Membrane: A thicker membrane increases the distance gases must travel, slowing down diffusion. Conditions like pulmonary edema (fluid accumulation in the lungs) or pneumonia (lung inflammation) can increase the membrane thickness and hinder gas exchange.
-
Partial Pressure Gradient: A larger difference in partial pressure between the alveoli and the blood accelerates diffusion. Conditions that reduce alveolar PO2, such as high altitude or hypoventilation, can decrease the driving force for oxygen diffusion.
-
Diffusion Coefficient: This factor reflects the ease with which a gas can move through a given medium. Oxygen and carbon dioxide have different diffusion coefficients, with CO2 diffusing more readily than O2. This difference is important in maintaining equilibrium.
-
Solubility of Gases: The solubility of gases in the blood also affects diffusion rates. CO2 is significantly more soluble in blood than oxygen, allowing for efficient CO2 transport even though its partial pressure gradient is smaller.
Oxygen Transport and Utilization
Once oxygen diffuses from the alveoli into the blood, it binds to hemoglobin, a protein found within red blood cells. Hemoglobin's remarkable ability to bind and transport oxygen is crucial for delivering oxygen throughout the body. The amount of oxygen bound to hemoglobin is influenced by several factors, including PO2, pH, temperature, and the concentration of 2,3-bisphosphoglycerate (2,3-BPG). This binding is reversible, allowing oxygen to be released to tissues where it is needed.
Hemoglobin's Role in Oxygen Transport
Hemoglobin's high affinity for oxygen at the high PO2 of the pulmonary capillaries ensures efficient oxygen uptake. As blood reaches the tissues, where PO2 is lower, oxygen is released from hemoglobin, allowing it to diffuse into the cells for cellular respiration. The efficiency of oxygen unloading from hemoglobin is further influenced by the metabolic demands of the tissues. Active tissues have lower pH and higher temperature, which promote oxygen release from hemoglobin, enhancing oxygen delivery where it is most needed.
Carbon Dioxide Transport and Elimination
Carbon dioxide, a byproduct of cellular respiration, diffuses from the tissues into the blood. Unlike oxygen, which is primarily transported bound to hemoglobin, carbon dioxide is transported in the blood in three main forms:
-
Dissolved in Plasma: A small portion of CO2 is dissolved directly in the blood plasma.
-
Bound to Hemoglobin: Some CO2 binds to hemoglobin, but with a lower affinity than oxygen. This binding occurs at different sites on the hemoglobin molecule than oxygen binding, allowing for simultaneous transport of both gases.
-
As Bicarbonate Ions: The majority of CO2 is transported as bicarbonate ions (HCO3-). Within red blood cells, an enzyme called carbonic anhydrase catalyzes the reaction between CO2 and water to form carbonic acid (H2CO3), which quickly dissociates into H+ and HCO3-. The bicarbonate ions diffuse out of the red blood cells into the plasma, while the H+ ions bind to hemoglobin, buffering the blood's pH.
Carbon Dioxide Elimination at the Alveoli
At the alveoli, the process reverses. The lower PCO2 in alveolar air drives CO2 diffusion from the blood into the alveoli. The bicarbonate ions are converted back to CO2 within red blood cells, facilitated by carbonic anhydrase, and then exhaled. This intricate mechanism ensures efficient elimination of CO2, preventing its accumulation in the blood, which could lead to acidosis.
Clinical Implications: When Gas Exchange Goes Wrong
Disruptions in the diffusion of respiratory gases can have serious consequences, leading to various respiratory and systemic disorders. Several diseases and conditions can impair gas exchange at the alveolar level:
-
Emphysema: Destruction of alveolar walls reduces the surface area available for gas exchange, leading to hypoxia (low blood oxygen levels) and hypercapnia (high blood carbon dioxide levels).
-
Pulmonary Edema: Fluid accumulation in the alveoli and interstitial spaces thickens the respiratory membrane, impairing diffusion. This can cause shortness of breath and reduced oxygen saturation.
-
Pneumonia: Inflammation and fluid buildup in the lungs hinder gas exchange, leading to hypoxia and respiratory distress.
-
Asthma: Bronchoconstriction (narrowing of the airways) reduces airflow to the alveoli, limiting gas exchange.
-
Pulmonary Fibrosis: Scarring and thickening of lung tissue impede gas exchange.
-
High Altitude Sickness: Reduced atmospheric pressure at high altitudes lowers the alveolar PO2, leading to hypoxia.
Conclusion: A Vital Process
The diffusion of respiratory gases at the alveoli is a fundamental process that sustains life. The intricate structure of the alveoli, the principles of diffusion, and the sophisticated mechanisms of oxygen and carbon dioxide transport all contribute to the remarkable efficiency of this process. Understanding the mechanics of pulmonary gas exchange is crucial for diagnosing and managing a wide range of respiratory disorders and ensuring optimal respiratory health. Future research continues to refine our understanding of this complex process, leading to improved diagnostic tools and therapeutic interventions.
Latest Posts
Latest Posts
-
Are Humans Hot Blooded Or Cold Blooded
Apr 19, 2025
-
Baking A Cake Physical Or Chemical Change
Apr 19, 2025
-
Battery In Series And Parallel Formula
Apr 19, 2025
-
What Is A 40 Out Of 60
Apr 19, 2025
-
Greatest Common Factor Of 12 And 20
Apr 19, 2025
Related Post
Thank you for visiting our website which covers about Diffusion Of Respiratory Gases Takes Place At The . We hope the information provided has been useful to you. Feel free to contact us if you have any questions or need further assistance. See you next time and don't miss to bookmark.