Describe The Shape Of The Dna Molecule
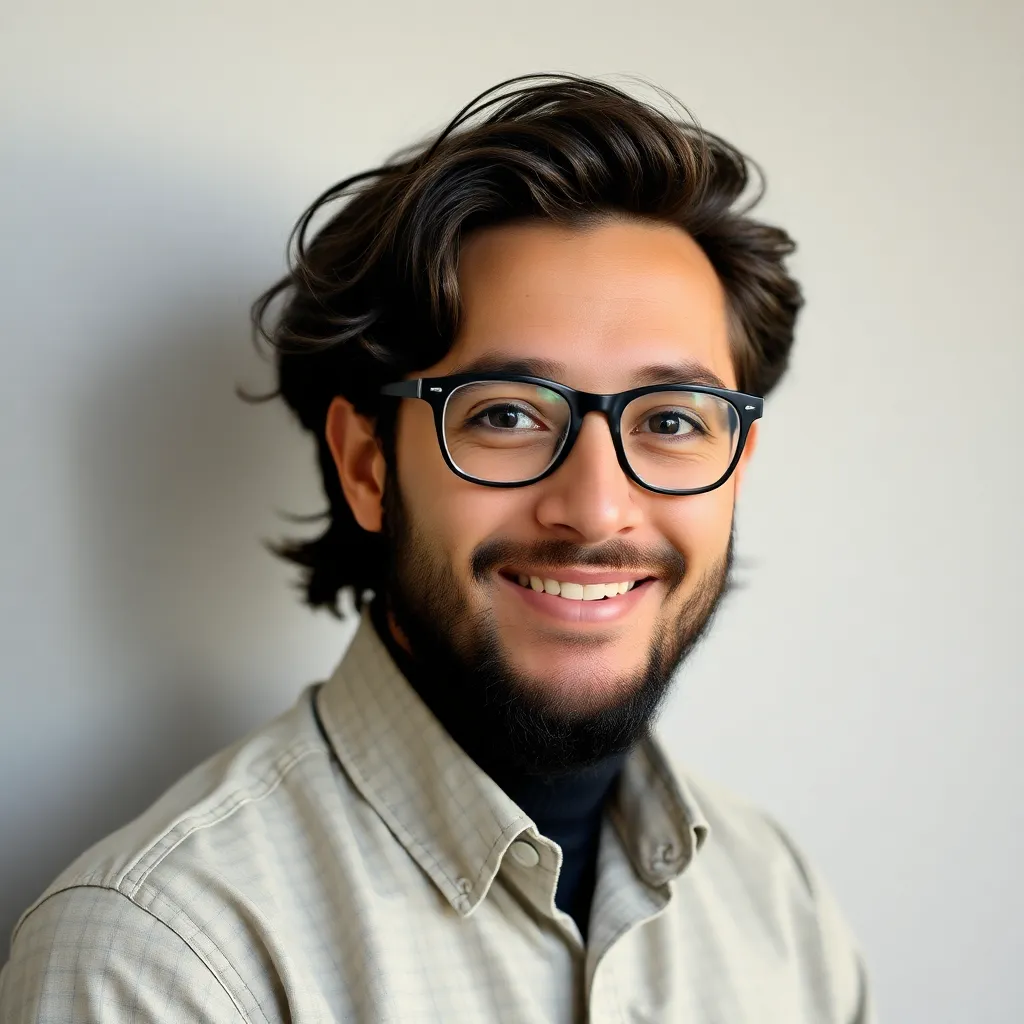
Juapaving
Apr 15, 2025 · 7 min read

Table of Contents
- Describe The Shape Of The Dna Molecule
- Table of Contents
- Decoding the Double Helix: A Deep Dive into the Shape of the DNA Molecule
- The Building Blocks: Nucleotides and their Roles
- The Double Helix: A Twist on the Molecular Scale
- Key Features of the Double Helix:
- The Significance of the Double Helix Shape
- Beyond the Classic B-DNA: Variations in DNA Structure
- Studying DNA Shape: Techniques and Advancements
- Conclusion: The Enduring Significance of DNA's Shape
- Latest Posts
- Latest Posts
- Related Post
Decoding the Double Helix: A Deep Dive into the Shape of the DNA Molecule
The shape of the DNA molecule, a breathtaking double helix, is arguably one of the most iconic and significant discoveries in the history of science. Understanding this shape is crucial to grasping how DNA functions – how it replicates, how it carries genetic information, and how it interacts with other molecules within the cell. This article will delve into the intricate details of the DNA molecule's structure, exploring its components, its overall form, and the implications of this specific shape for life as we know it.
The Building Blocks: Nucleotides and their Roles
Before delving into the overall shape, let's understand the fundamental components: nucleotides. These are the monomers that form the polymeric DNA chain. Each nucleotide consists of three parts:
-
A deoxyribose sugar: This five-carbon sugar provides the backbone structure of the DNA molecule. The "deoxy" prefix signifies that it lacks an oxygen atom compared to ribose, the sugar found in RNA. This subtle difference has significant implications for the stability and function of DNA.
-
A phosphate group: This negatively charged group links adjacent deoxyribose sugars, creating the sugar-phosphate backbone. The negative charge is crucial for DNA's interaction with proteins and its overall stability. The phosphate groups link the 3' carbon of one sugar to the 5' carbon of the next, establishing the 5' to 3' directionality of the DNA strand.
-
A nitrogenous base: This is the part of the nucleotide that carries the genetic information. There are four types of nitrogenous bases in DNA: adenine (A), guanine (G), cytosine (C), and thymine (T). These bases are categorized as either purines (A and G, which have a double-ring structure) or pyrimidines (C and T, which have a single-ring structure). The sequence of these bases along the DNA strand determines the genetic code.
The Double Helix: A Twist on the Molecular Scale
The individual nucleotides don't exist in isolation; they are joined together to form long polynucleotide chains. These chains aren't just linear; they are twisted into a double helix, a structure resembling a spiraling ladder. This remarkable shape is crucial for DNA's function.
Key Features of the Double Helix:
-
Two antiparallel strands: The two polynucleotide chains run in opposite directions. One strand runs 5' to 3', while the other runs 3' to 5'. This antiparallel arrangement is critical for DNA replication and transcription.
-
Base pairing: The nitrogenous bases of the two strands are paired together through hydrogen bonds. Adenine (A) always pairs with thymine (T) via two hydrogen bonds, while guanine (G) always pairs with cytosine (C) via three hydrogen bonds. This specific base pairing, known as Chargaff's rules, ensures that the width of the double helix remains consistent throughout. The stronger G-C base pairs contribute to regions of DNA that are more resistant to denaturation (separation of the strands).
-
Major and minor grooves: The double helix isn't uniformly smooth; it has grooves of varying widths. The major groove is wider than the minor groove. These grooves provide access points for proteins to bind to the DNA, allowing them to read the genetic code and regulate gene expression. The difference in groove width allows proteins to recognize specific base sequences without needing to fully unwind the helix.
-
Helical parameters: The double helix isn't a perfect, uniform spiral. Several parameters describe its geometry, including the diameter (approximately 2 nanometers), the pitch (the distance it takes to complete one full turn, approximately 3.4 nanometers), and the number of base pairs per turn (approximately 10). These parameters can vary slightly depending on factors such as the DNA sequence and the surrounding environment. Variations in these parameters can influence DNA's flexibility and its interaction with other molecules.
-
Supercoiling: In cells, the DNA molecule is often further organized into structures called supercoils. This compacting is achieved through twisting the double helix upon itself, allowing vast lengths of DNA to be packaged into the confined space of a cell's nucleus. The degree of supercoiling is regulated by enzymes called topoisomerases, which influence DNA's accessibility for replication and transcription. The type of supercoiling (positive or negative) also has functional implications.
The Significance of the Double Helix Shape
The double helix shape is far more than just an aesthetically pleasing structure; it's essential for DNA's function:
-
Replication: The double helix allows for straightforward DNA replication. The two strands separate, and each serves as a template for the synthesis of a new complementary strand. The antiparallel nature of the strands ensures that the new strands are synthesized in the correct 5' to 3' direction.
-
Transcription: The double helix structure also facilitates transcription, the process of copying the DNA sequence into RNA. The unwinding of the double helix allows RNA polymerase to access the DNA template strand and synthesize a complementary RNA molecule.
-
Genetic information storage: The sequence of bases along the DNA strands encodes the genetic information. The double-stranded nature of the molecule provides redundancy, protecting the genetic information from damage. If one strand is damaged, the other strand can serve as a template for repair.
-
Regulation of gene expression: The major and minor grooves of the double helix provide binding sites for proteins that regulate gene expression. These proteins can either activate or repress the transcription of specific genes, controlling which genes are expressed at any given time. The subtle differences in groove width and the specific sequences exposed in the grooves determine protein binding specificity.
Beyond the Classic B-DNA: Variations in DNA Structure
While the B-DNA form (the classic double helix) is the most common form found in cells under physiological conditions, DNA can adopt other conformations depending on environmental factors like hydration, ion concentration, and sequence composition. These alternative forms include:
-
A-DNA: A-DNA is a right-handed helix, but it's shorter and wider than B-DNA. It's often found in dehydrated conditions.
-
Z-DNA: Z-DNA is a left-handed helix with a zigzag pattern. Its formation is influenced by specific DNA sequences and is implicated in gene regulation.
-
Other non-B forms: Several other less common DNA structures exist, often associated with specific sequences or regions of the genome, which may play roles in DNA replication, transcription, or recombination. These less common forms highlight the flexibility of the DNA molecule and its capacity to adapt to different conditions.
Studying DNA Shape: Techniques and Advancements
Scientists have employed various techniques to study the shape of the DNA molecule:
-
X-ray diffraction: The iconic image of the DNA double helix was produced using X-ray diffraction, a technique that reveals the molecule's overall structure.
-
Nuclear magnetic resonance (NMR) spectroscopy: NMR provides detailed information about the molecule's three-dimensional structure at the atomic level.
-
Cryo-electron microscopy (cryo-EM): Cryo-EM allows for the visualization of large DNA complexes, providing insights into how DNA interacts with other molecules. The development of advanced cryo-EM techniques has significantly improved our understanding of DNA's structure and dynamics.
-
Computational modeling: Computer simulations are used to model DNA's behavior under various conditions, providing insights into its dynamics and interactions. These models aid in understanding how DNA's shape changes under different environmental stresses.
Conclusion: The Enduring Significance of DNA's Shape
The discovery of the DNA double helix revolutionized biology. Understanding its shape is fundamental to understanding life itself. The double helix's intricate structure, with its precise base pairing, antiparallel strands, and major and minor grooves, underpins the molecule's ability to store, replicate, and express genetic information. Ongoing research continues to unveil the complexities of DNA structure, revealing subtle variations and interactions that play essential roles in cellular processes. The double helix remains an iconic symbol, representing not just a remarkable scientific discovery but the very foundation of life. Its continued study promises further insights into the intricacies of life's processes and the development of new therapeutic strategies.
Latest Posts
Latest Posts
-
What Organelle Is Found Only In Animal Cells
Apr 20, 2025
-
What Are The First Five Multiples Of 4
Apr 20, 2025
-
Human Somatic Cells Contain How Many Chromosomes
Apr 20, 2025
-
40 Is What Percent Of 200
Apr 20, 2025
-
Does Your Hair Grow When Your Dead
Apr 20, 2025
Related Post
Thank you for visiting our website which covers about Describe The Shape Of The Dna Molecule . We hope the information provided has been useful to you. Feel free to contact us if you have any questions or need further assistance. See you next time and don't miss to bookmark.