Converts Chemical Energy To Electrical Energy
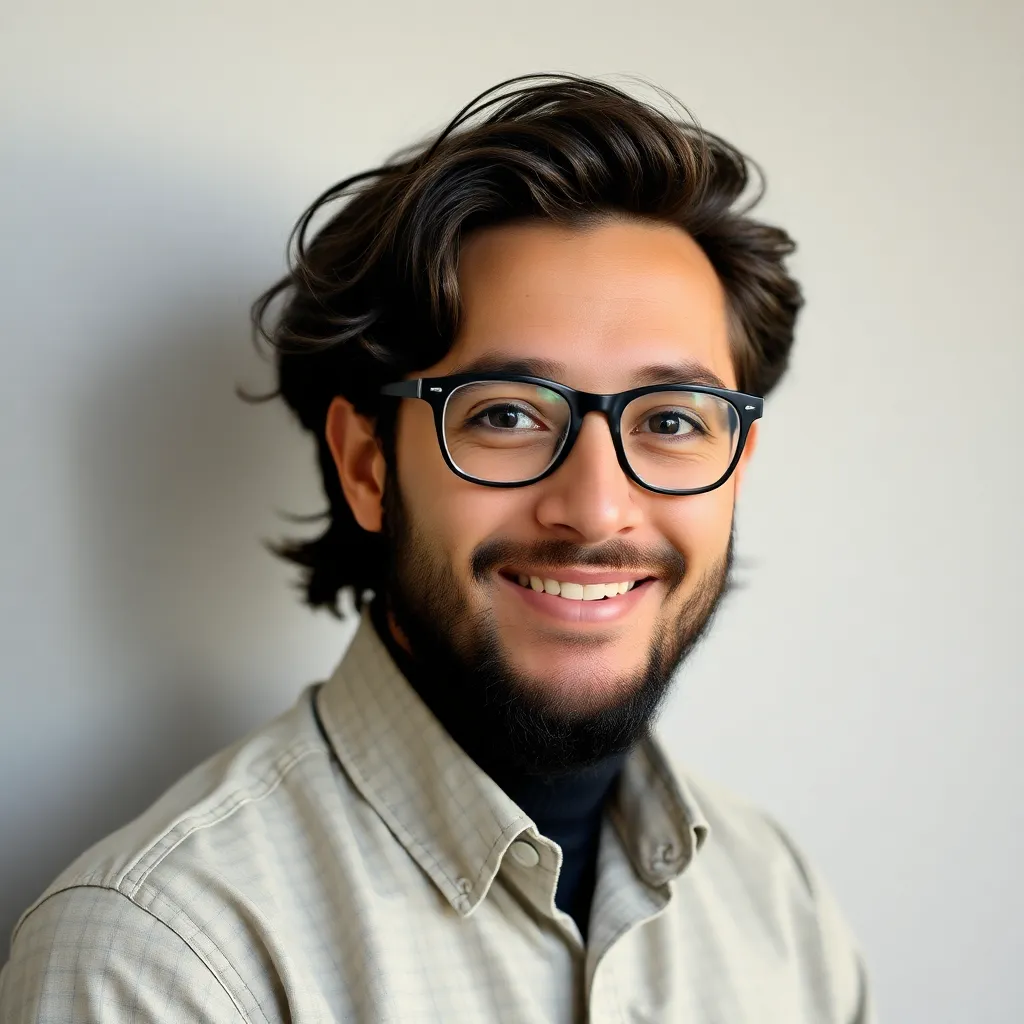
Juapaving
Apr 25, 2025 · 6 min read

Table of Contents
Converting Chemical Energy to Electrical Energy: A Deep Dive into Electrochemical Processes
The conversion of chemical energy into electrical energy is a fundamental process underlying many technologies we rely on daily. From the batteries powering our smartphones to the fuel cells propelling electric vehicles, this electrochemical transformation is crucial to modern life. This article delves into the mechanisms behind this conversion, exploring various methods, applications, and future directions in the field.
Understanding the Basics: Electrochemistry and Redox Reactions
At the heart of chemical-to-electrical energy conversion lies electrochemistry, the branch of chemistry dealing with the relationship between electrical energy and chemical change. This process hinges on redox reactions, or oxidation-reduction reactions, which involve the transfer of electrons between chemical species.
Oxidation is the loss of electrons, while reduction is the gain of electrons. These two processes are always coupled; one cannot occur without the other. In electrochemical cells, oxidation occurs at the anode, and reduction occurs at the cathode. This electron transfer creates a flow of electrons, generating an electric current.
Types of Electrochemical Cells
Several types of electrochemical cells facilitate the conversion of chemical energy to electrical energy. The most common are:
-
Galvanic Cells (Voltaic Cells): These cells spontaneously generate electricity from a chemical reaction. The driving force is the difference in electrochemical potential between the two half-cells. Common examples include batteries found in everyday devices.
-
Electrolytic Cells: These cells require an external source of electrical energy to drive a non-spontaneous chemical reaction. This process is used in electroplating, electrolysis of water, and other industrial applications. Unlike galvanic cells, electrolytic cells consume electricity rather than producing it.
Batteries: A Ubiquitous Form of Chemical-to-Electrical Energy Conversion
Batteries are arguably the most prevalent example of chemical-to-electrical energy conversion. They are self-contained electrochemical cells that provide a portable source of electrical power. Several types of batteries exist, each with its own chemical composition, energy density, and lifespan:
1. Primary Batteries (Non-Rechargeable)
Primary batteries are designed for single use and cannot be recharged. Once the chemical reactants are consumed, the battery is depleted. Common examples include:
-
Zinc-Carbon Batteries: These are inexpensive and widely available but have relatively low energy density and a short lifespan.
-
Alkaline Batteries: These offer improved energy density and longer lifespan compared to zinc-carbon batteries, making them a popular choice for many applications.
-
Lithium-iron Disulfide (LiFeS2) Batteries: These are robust primary batteries with longer shelf lives and high energy density compared to alkaline, but costlier.
2. Secondary Batteries (Rechargeable)
Secondary batteries, also known as rechargeable batteries, can be recharged multiple times by reversing the electrochemical reaction. This makes them environmentally friendly and cost-effective in the long run. Common types include:
-
Lead-Acid Batteries: These are heavy and bulky but offer high current output, making them suitable for automotive applications.
-
Nickel-Cadmium (NiCd) Batteries: These were once popular but have largely been replaced by nickel-metal hydride batteries due to environmental concerns regarding cadmium toxicity.
-
Nickel-Metal Hydride (NiMH) Batteries: These offer higher energy density than NiCd batteries and are environmentally friendlier.
-
Lithium-ion Batteries (Li-ion): These are currently the dominant rechargeable battery technology, boasting high energy density, long lifespan, and relatively low self-discharge rate. They power a wide range of portable electronic devices and electric vehicles. Various chemistries exist within Li-ion batteries, including Lithium Cobalt Oxide (LCO), Lithium Manganese Oxide (LMO), Lithium Nickel Manganese Cobalt Oxide (NMC), and Lithium Iron Phosphate (LFP), each offering a unique balance of performance and safety characteristics.
-
Solid-State Batteries: This emerging technology replaces the liquid or gel electrolyte with a solid electrolyte. Solid-state batteries offer potential advantages in terms of safety, energy density, and lifespan, but face challenges in terms of manufacturing and cost.
Fuel Cells: Continuous Chemical-to-Electrical Energy Conversion
Fuel cells differ from batteries in that they continuously convert chemical energy into electrical energy as long as fuel and oxidant are supplied. They are not rechargeable in the traditional sense; they simply require refueling. The most common type is the proton exchange membrane (PEM) fuel cell, which uses hydrogen as fuel and oxygen as the oxidant.
How PEM Fuel Cells Work
In a PEM fuel cell, hydrogen gas is fed to the anode, where it undergoes oxidation, releasing electrons and protons. The electrons flow through an external circuit, generating electricity. The protons pass through a proton exchange membrane (PEM) to the cathode, where they combine with oxygen and the electrons to produce water.
Advantages and Disadvantages of Fuel Cells
Fuel cells offer several advantages over batteries, including:
- Higher energy density: They can generate more power per unit volume or weight.
- Continuous power generation: They can operate continuously as long as fuel is supplied.
- Zero or low emissions: PEM fuel cells produce only water as a byproduct.
However, fuel cells also have some drawbacks:
- High cost: The manufacturing cost of fuel cells is currently high.
- Fuel storage and transportation: Storing and transporting hydrogen fuel can be challenging.
- Durability and lifespan: The lifespan of fuel cells can be limited by degradation of the membrane and other components.
Other Methods of Chemical-to-Electrical Energy Conversion
Beyond batteries and fuel cells, several other methods exist for converting chemical energy into electrical energy:
-
Thermoelectric Generators: These devices convert heat energy into electrical energy using the Seebeck effect. While not directly converting chemical energy, they can be coupled with combustion processes to generate electricity from chemical fuels.
-
Piezoelectric Devices: These devices generate electricity from mechanical stress or pressure. While not directly converting chemical energy, some chemical reactions can produce mechanical stress, enabling indirect energy conversion.
-
Biofuel Cells: These cells utilize biological systems, such as enzymes or microorganisms, to catalyze redox reactions and generate electricity. They show promise for powering implantable medical devices and other applications requiring low-power sources.
Future Directions in Chemical-to-Electrical Energy Conversion
Research and development in chemical-to-electrical energy conversion are continually pushing boundaries. Key areas of focus include:
-
Improved Battery Technology: Efforts are underway to develop batteries with higher energy density, longer lifespan, faster charging times, and improved safety. Solid-state batteries, lithium-sulfur batteries, and other advanced battery chemistries are promising candidates.
-
Advanced Fuel Cell Technologies: Research is focused on developing more efficient and cost-effective fuel cells, including high-temperature fuel cells and direct methanol fuel cells.
-
Hybrid and Integrated Systems: Combining different energy conversion technologies, such as fuel cells and batteries, can create more efficient and versatile energy systems.
-
Sustainable and Renewable Fuels: Developing sustainable fuels, such as biofuels and hydrogen produced from renewable energy sources, is crucial for environmentally friendly chemical-to-electrical energy conversion.
Conclusion
The conversion of chemical energy into electrical energy is a cornerstone of modern technology, powering our devices, vehicles, and countless other applications. While batteries and fuel cells are currently the dominant technologies, ongoing research and development promise even more efficient, sustainable, and powerful methods for harnessing the energy stored within chemical bonds. The future of energy conversion will likely involve a diverse portfolio of technologies, each tailored to specific applications and requirements, driving innovation and shaping a more sustainable energy landscape.
Latest Posts
Latest Posts
-
The Two Main Types Of Fermentation Are Called
Apr 25, 2025
-
Five Letter Words Ending With En
Apr 25, 2025
-
Label The Parts Of Female Reproductive System
Apr 25, 2025
-
What Are The Units Of Energy
Apr 25, 2025
-
What Organelle Is Dna Found In
Apr 25, 2025
Related Post
Thank you for visiting our website which covers about Converts Chemical Energy To Electrical Energy . We hope the information provided has been useful to you. Feel free to contact us if you have any questions or need further assistance. See you next time and don't miss to bookmark.