Color By Number Molecular Geometry And Polarity
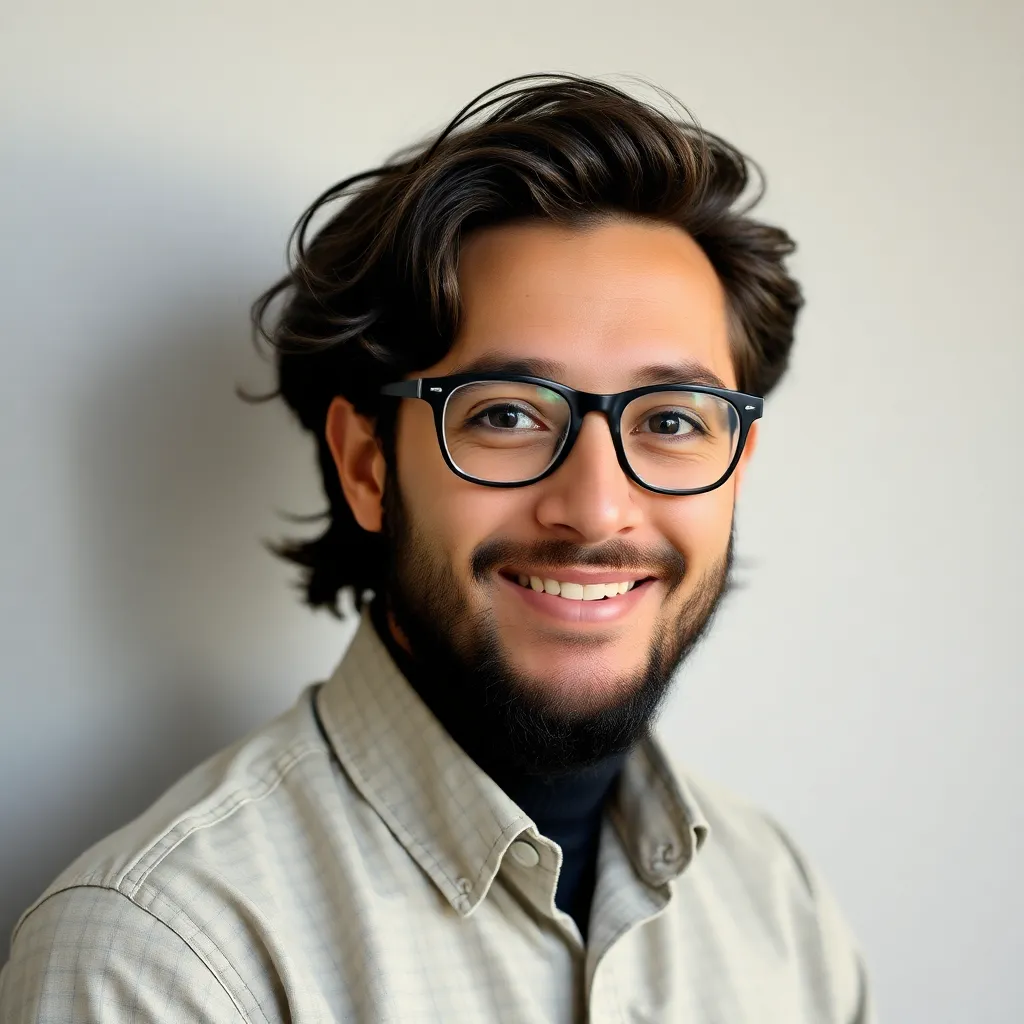
Juapaving
May 24, 2025 · 7 min read
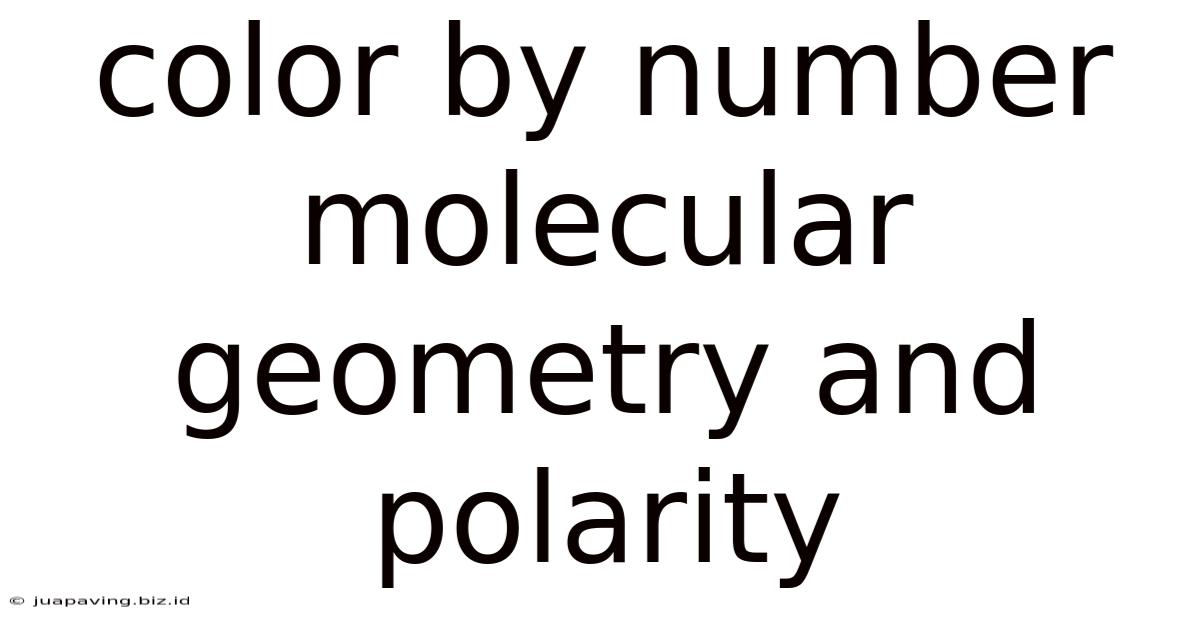
Table of Contents
Color by Number: Molecular Geometry and Polarity
Understanding molecular geometry and polarity is fundamental to grasping the behavior of molecules. These concepts are crucial in various fields, from chemistry and biology to materials science and pharmacology. While textbooks often present these topics with complex diagrams and equations, a more intuitive approach can greatly enhance understanding. This article employs a "color-by-number" analogy to illustrate molecular geometry and polarity, simplifying these complex concepts and making them more accessible.
What is Molecular Geometry?
Molecular geometry refers to the three-dimensional arrangement of atoms within a molecule. It's not just about the bonds between atoms; it's about the overall spatial structure, including bond angles and the relative positions of atoms in space. This arrangement significantly influences a molecule's properties, including its reactivity, polarity, and physical state.
Think of it like building with LEGOs. You have different types of LEGO bricks (atoms) and instructions (chemical formula) telling you how to connect them. The final structure you build represents the molecule's geometry. Different building instructions (different molecules) lead to different structures (different geometries).
VSEPR Theory: The "Coloring Book" of Molecular Geometry
The Valence Shell Electron Pair Repulsion (VSEPR) theory is the primary tool used to predict molecular geometry. VSEPR theory dictates that electron pairs (both bonding and non-bonding) around a central atom will arrange themselves to minimize repulsion. This principle helps us "color" the molecule's structure based on the number of electron domains around the central atom.
-
Electron Domains: These include both bonding pairs (electrons shared between atoms) and lone pairs (non-bonding electrons on the central atom).
-
Minimizing Repulsion: Electrons, being negatively charged, repel each other. They arrange themselves as far apart as possible to minimize this repulsion, determining the molecule's geometry.
Imagine each electron domain as a differently colored spot on your "coloring sheet." The arrangement of these spots determines the overall shape.
Common Molecular Geometries: A Color-Coded Guide
Number of Electron Domains | Electron Domain Geometry | Molecular Geometry (Examples) | Description |
---|---|---|---|
2 | Linear | BeCl₂ , CO₂ | Atoms arranged in a straight line. |
3 | Trigonal Planar | BF₃, SO₃ | Atoms arranged in a flat triangle. |
3 (2 bonds, 1 lone pair) | Bent | SO₂, H₂O | Similar to trigonal planar, but the lone pair pushes the bonding pairs closer. |
4 | Tetrahedral | CH₄, CCl₄ | Atoms arranged in a three-dimensional tetrahedron (pyramid with triangular base). |
4 (3 bonds, 1 lone pair) | Trigonal Pyramidal | NH₃, PH₃ | Similar to tetrahedral, but the lone pair distorts the shape. |
4 (2 bonds, 2 lone pairs) | Bent | H₂O, O₃ | Significant distortion due to two lone pairs. |
5 | Trigonal Bipyramidal | PCl₅ | Three atoms in a plane, with two atoms above and below. |
5 (4 bonds, 1 lone pair) | See-Saw | SF₄ | Distorted trigonal bipyramidal due to lone pair. |
5 (3 bonds, 2 lone pairs) | T-Shaped | ClF₃ | Lone pairs further distort the shape into a T. |
5 (2 bonds, 3 lone pairs) | Linear | XeF₂ | Lone pairs force the bonding pairs into a linear arrangement. |
6 | Octahedral | SF₆ | Atoms arranged around the central atom in an octahedron (two square pyramids joined at their bases). |
6 (5 bonds, 1 lone pair) | Square Pyramidal | BrF₅ | Distorted octahedral due to lone pair. |
6 (4 bonds, 2 lone pairs) | Square Planar | XeF₄ | Lone pairs cause a significant distortion into a square plane. |
What is Molecular Polarity?
Molecular polarity refers to the overall distribution of charge within a molecule. A molecule is considered polar if it has a dipole moment, meaning it has a slightly positive end and a slightly negative end. This dipole moment arises from the difference in electronegativity between atoms.
Electronegativity is the ability of an atom to attract electrons towards itself in a chemical bond. When atoms with different electronegativities bond, the electrons are not shared equally, resulting in a partial positive charge (δ+) on the less electronegative atom and a partial negative charge (δ-) on the more electronegative atom.
Imagine this as coloring the molecule with two colors: one for partially positive regions (δ+) and one for partially negative regions (δ-). If the colors are distributed unevenly, the molecule is polar.
Predicting Molecular Polarity: Combining Geometry and Electronegativity
Molecular polarity depends on both the molecular geometry and the electronegativity difference between atoms.
-
Symmetrical Molecules: If a molecule is symmetrical and all its bonds are nonpolar (atoms with similar electronegativity), the molecule is nonpolar. The individual bond dipoles cancel each other out. Think of it like perfectly balanced colors – the overall effect is neutral.
-
Asymmetrical Molecules: If a molecule is asymmetrical, or if it has polar bonds, the molecule is likely polar. The individual bond dipoles do not cancel each other out, resulting in an overall dipole moment. The colors are unevenly distributed, leading to a noticeable polarity.
Examples: Putting it all Together
Let's illustrate with examples:
-
CO₂ (Carbon Dioxide): CO₂ has a linear geometry. Oxygen is more electronegative than carbon, creating polar bonds. However, due to the symmetrical linear structure, the bond dipoles cancel each other out, making the molecule nonpolar.
-
H₂O (Water): H₂O has a bent geometry. Oxygen is more electronegative than hydrogen, creating polar bonds. The bent shape prevents the bond dipoles from canceling each other out, resulting in a net dipole moment, making water a polar molecule.
-
CH₄ (Methane): CH₄ has a tetrahedral geometry. The electronegativity difference between carbon and hydrogen is small, resulting in nearly nonpolar bonds. The symmetrical tetrahedral structure ensures the bond dipoles cancel out, making methane nonpolar.
-
NH₃ (Ammonia): NH₃ has a trigonal pyramidal geometry. Nitrogen is more electronegative than hydrogen, creating polar bonds. The asymmetrical shape results in a net dipole moment, making ammonia a polar molecule.
Applications of Molecular Geometry and Polarity
Understanding molecular geometry and polarity is critical in numerous scientific disciplines:
-
Solubility: Polar molecules tend to dissolve in polar solvents (like water), while nonpolar molecules dissolve in nonpolar solvents (like oil). This principle is fundamental to understanding chemical reactions and biological processes.
-
Boiling and Melting Points: Polar molecules generally have higher boiling and melting points than nonpolar molecules of comparable size due to stronger intermolecular forces (dipole-dipole interactions and hydrogen bonding).
-
Reactivity: Molecular geometry and polarity influence the reactivity of molecules, determining how they interact with other molecules and participate in chemical reactions.
-
Biological Systems: The polarity of molecules plays a crucial role in biological systems. For example, the polarity of water is essential for its role as a solvent in biological processes, while the polarity of proteins and lipids influences their structure and function.
-
Materials Science: Understanding molecular geometry and polarity is essential in designing new materials with specific properties, such as conductivity, strength, and reactivity.
Advanced Concepts: Beyond the Basics
While the "color-by-number" analogy simplifies the concepts, several advanced topics add further complexity:
-
Hybridization: This concept explains the mixing of atomic orbitals to form hybrid orbitals, which better describe the bonding in molecules.
-
Molecular Orbital Theory: This theory offers a more sophisticated view of bonding, considering the interactions of atomic orbitals to form molecular orbitals.
-
Intermolecular Forces: These forces exist between molecules and include dipole-dipole interactions, hydrogen bonding, and London dispersion forces. These forces are significantly influenced by molecular polarity and geometry.
Conclusion: Mastering the Art of Molecular Structure
Molecular geometry and polarity are fundamental concepts in chemistry with wide-ranging applications. By using visual analogies and breaking down complex theories into manageable steps, we can develop a deeper understanding of how these concepts dictate the properties and behavior of molecules. The "color-by-number" approach presented here offers a simplified yet effective method to grasp these vital aspects of molecular structure and reactivity. Remember, practice is key. Work through numerous examples, applying the VSEPR theory and electronegativity principles to predict the geometry and polarity of various molecules. The more you practice, the more proficient you will become at visualizing and understanding the intricate world of molecular structures.
Latest Posts
Latest Posts
-
Identifying Transformations Homework 5 Answer Key
May 24, 2025
-
Animal Farm The Battle Of The Windmill
May 24, 2025
-
Homework 6 Parts Of Similar Triangles
May 24, 2025
-
Translate The Medical Term Hypoglycemic As Literally As Possible
May 24, 2025
-
The End Of Something By Ernest Hemingway
May 24, 2025
Related Post
Thank you for visiting our website which covers about Color By Number Molecular Geometry And Polarity . We hope the information provided has been useful to you. Feel free to contact us if you have any questions or need further assistance. See you next time and don't miss to bookmark.