Cardiac Action Potential Phases 0 4
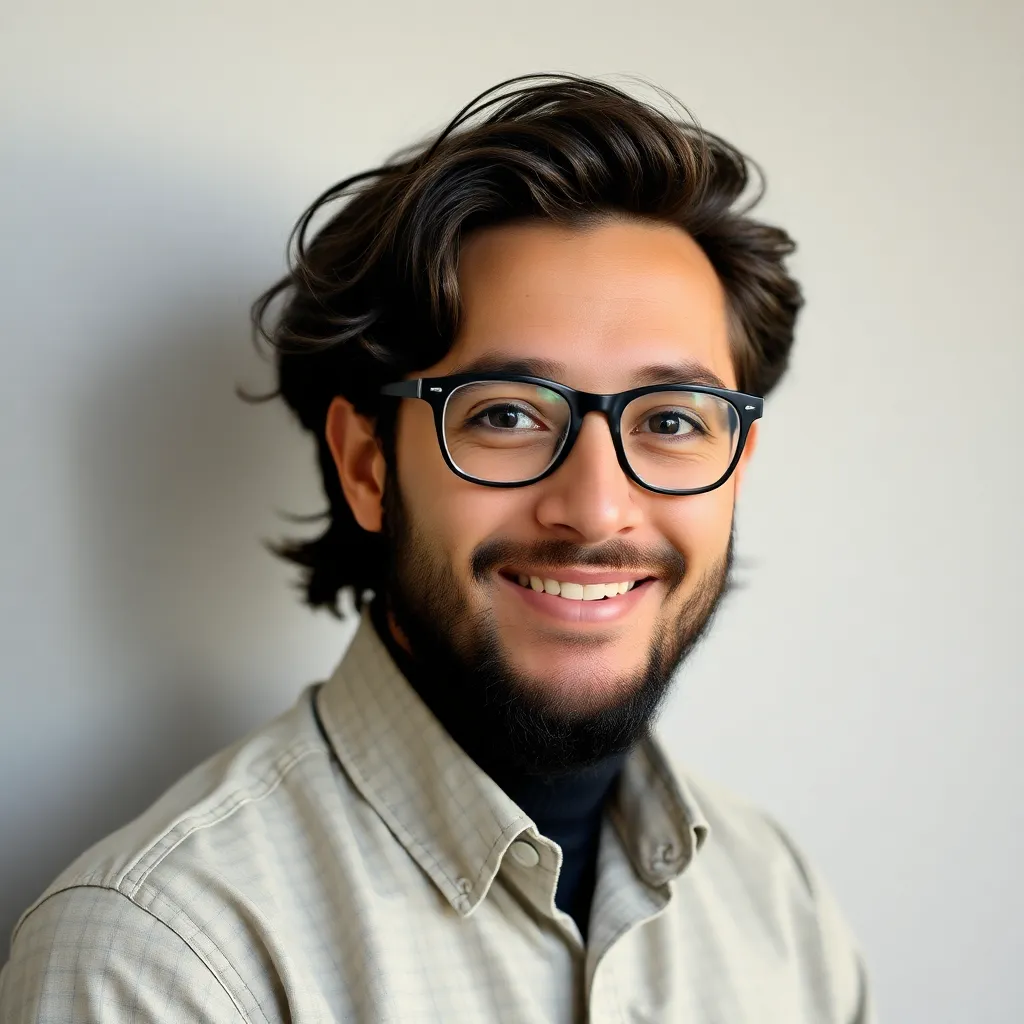
Juapaving
May 11, 2025 · 6 min read
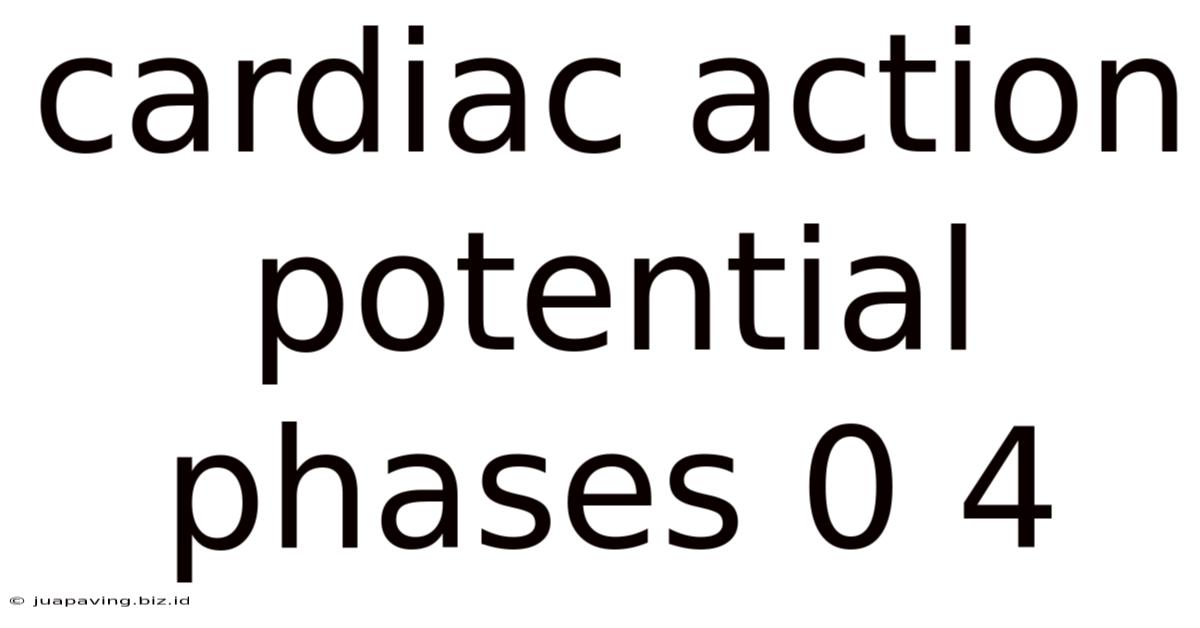
Table of Contents
Cardiac Action Potential: A Deep Dive into Phases 0 and 4
The heart, a tireless engine driving life, relies on the intricate dance of electrical signals to orchestrate its rhythmic contractions. These electrical signals, manifested as cardiac action potentials, are the cornerstone of cardiac function. Understanding their phases is crucial for grasping the mechanics of the heartbeat and the complexities of cardiac arrhythmias. This article will delve into the crucial phases 0 and 4 of the cardiac action potential, providing a comprehensive overview of their ionic mechanisms and physiological significance.
Phase 0: Rapid Depolarization – The Upstroke of Excitation
Phase 0, the rapid depolarization phase, marks the beginning of the action potential. This dramatic upswing in membrane potential is characterized by a swift influx of sodium ions (Na+) into the cardiomyocyte. The driving force behind this rapid depolarization is the opening of voltage-gated sodium channels. These channels, highly selective for Na+, are activated when the membrane potential reaches a critical threshold, typically around -65 mV.
The Role of Voltage-Gated Sodium Channels
The voltage-gated sodium channels are complex molecular machines that exist in different conformations: closed, open, and inactivated. At resting membrane potential, these channels are predominantly in the closed state. Upon reaching the threshold potential, a conformational change opens the channels, creating a pore that allows Na+ ions to rush into the cell along their electrochemical gradient. This influx of positively charged ions dramatically increases the membrane potential, leading to the characteristic steep upstroke of Phase 0.
The Significance of Steepness
The steepness of Phase 0 is critical. It ensures rapid and efficient propagation of the action potential throughout the heart. A less steep depolarization would lead to slower conduction velocity, potentially disrupting the coordinated contraction of the heart. The rate of depolarization, measured as the maximum rate of rise (dV/dt), is clinically significant and is often assessed in electrocardiograms (ECGs) to evaluate cardiac function. Reduced dV/dt can indicate conditions like myocardial infarction (heart attack) where the sodium channels function is compromised.
Inactivation of Sodium Channels
Importantly, the voltage-gated sodium channels rapidly inactivate after opening. This inactivation, a crucial self-limiting mechanism, prevents the sustained influx of Na+ and is vital for the repolarization process. The inactivation process is crucial for the refractory period, a period of time during which the cell is unable to generate another action potential, preventing potentially fatal arrhythmias.
Phase 4: Resting Membrane Potential – The Silent Interlude
Phase 4 represents the resting membrane potential of the cardiomyocyte. This is a relatively stable period of low membrane potential, typically between -90 mV and -70 mV, during which the cell is electrically quiescent. However, this "resting" state isn't truly inactive; it's a dynamic equilibrium maintained by a delicate balance of ionic currents.
The Role of Potassium Channels
The primary factor maintaining the resting membrane potential is the outward current of potassium ions (K+). Various potassium channels contribute to this outward current, including the inward rectifier potassium channels (IK1) and other potassium channels with different activation kinetics. IK1 channels are particularly important in maintaining the resting potential, as they are open at negative potentials and allow a slow but steady outward flow of K+. This outward flow of positive charge makes the inside of the cell more negative.
The Sodium-Potassium Pump
The sodium-potassium pump (Na+/K+-ATPase) actively transports three sodium ions out of the cell and two potassium ions into the cell for every molecule of ATP hydrolyzed. This pump plays a crucial role in maintaining the resting membrane potential by removing excess intracellular Na+ and replenishing intracellular K+. It is an energy-consuming process, vital for restoring ionic gradients disrupted during the action potential.
Spontaneous Depolarization in Pacemaker Cells
It is crucial to distinguish between the Phase 4 of ventricular cardiomyocytes and pacemaker cells (sinoatrial and atrioventricular nodes). In ventricular cardiomyocytes, Phase 4 is indeed a relatively flat, stable resting potential. However, in pacemaker cells, Phase 4 is characterized by a slow, spontaneous depolarization. This spontaneous depolarization, also known as the pacemaker potential, is driven by a unique set of ionic currents. These currents, including the funny current (If), a mixed sodium and potassium current, and calcium channels, cause a gradual increase in membrane potential until it reaches threshold, triggering a new action potential. This spontaneous depolarization is the driving force behind the heart's intrinsic rhythmicity.
The Importance of Calcium Influx in Phase 4 of Pacemaker Cells
In pacemaker cells, the gradual depolarization in Phase 4 is partly driven by calcium influx. The L-type calcium channels open during the latter part of Phase 4, contributing to the gradual increase in membrane potential. This influx of calcium ions is crucial for the pacemaker potential and therefore for the heart's ability to initiate rhythmic contractions. The balance between the outward potassium current and the inward calcium and funny current determines the rate of depolarization and, hence, the heart rate.
Clinical Implications of Phase 4 Alterations
Disruptions in Phase 4 can have significant clinical consequences. Conditions like bradycardia (slow heart rate) and heart blocks can result from impaired pacemaker activity due to alterations in the ionic currents underlying the spontaneous depolarization. Conversely, accelerated Phase 4 depolarization can lead to tachycardia (rapid heart rate). Understanding the ionic mechanisms of Phase 4 is vital for the diagnosis and management of these arrhythmias.
The Interplay Between Phases 0 and 4: Maintaining Cardiac Rhythm
Phases 0 and 4 are intimately linked in maintaining the heart's rhythmic contractions. The rapid depolarization of Phase 0 initiates the contraction, while the resting potential of Phase 4 provides the baseline for the next cycle. The duration of Phase 4, the time between consecutive action potentials, determines the heart rate.
The Role of the Refractory Period
The refractory period, the period following Phase 0 during which the cell is unresponsive to further stimulation, is crucial for preventing rapid, uncoordinated contractions. The refractory period ensures that the heart can effectively fill with blood between contractions. The duration of the refractory period is influenced by the ionic currents involved in repolarization.
The Impact of Electrolyte Imbalances
Electrolyte imbalances, particularly those involving potassium and calcium, can significantly impact both Phase 0 and Phase 4. Hypokalemia (low potassium) can prolong Phase 4 depolarization in pacemaker cells, leading to an increased heart rate, while hyperkalemia (high potassium) can shorten Phase 0 and diminish its steepness, potentially leading to heart block. Similarly, calcium imbalances can affect both the rate of depolarization and the resting membrane potential.
Conclusion: Understanding Cardiac Action Potentials for Better Healthcare
A thorough understanding of the cardiac action potential, especially its key phases like 0 and 4, is fundamental to comprehending normal cardiac function and the pathophysiology of many cardiac arrhythmias. The precise interplay of ion channels and their associated currents dictates the shape and duration of these phases, ultimately determining the heart's rhythm and contractile ability. Research into these mechanisms continues to unveil new insights into the complexities of the heart, leading to improved diagnostic techniques and treatment strategies for various cardiac diseases. Further exploration into the nuances of these phases will continue to drive advancements in cardiovascular medicine, offering hope for improved patient outcomes and enhanced cardiac health management.
Latest Posts
Latest Posts
-
Unit Of Account Function Of Money
May 12, 2025
-
Which Of The Following Is A Non Renewable Resources
May 12, 2025
-
The Intensity Of Sound Is Measured In This Unit
May 12, 2025
-
Label The Parts Of Male Reproductive System
May 12, 2025
-
Least Common Denominator Of 10 And 15
May 12, 2025
Related Post
Thank you for visiting our website which covers about Cardiac Action Potential Phases 0 4 . We hope the information provided has been useful to you. Feel free to contact us if you have any questions or need further assistance. See you next time and don't miss to bookmark.