At The End Of The Electron Transport Chain
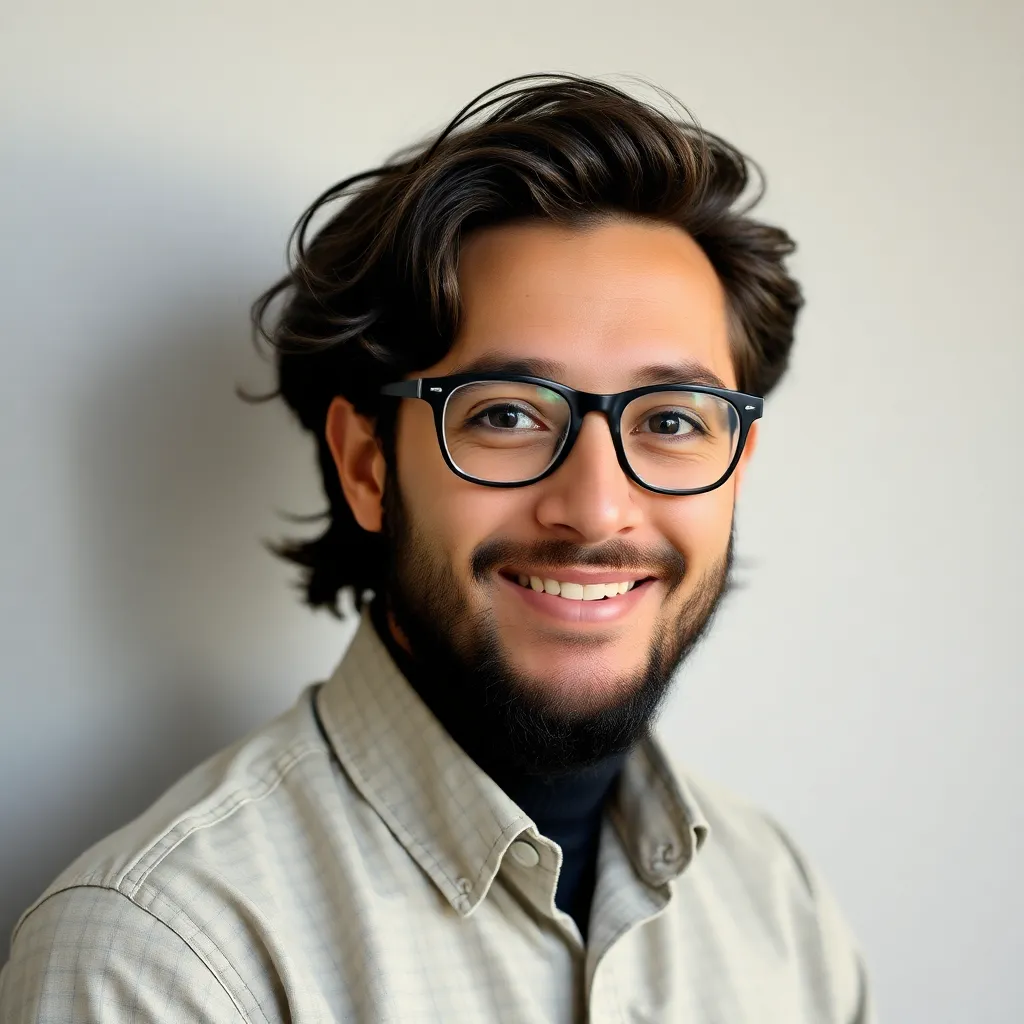
Juapaving
May 13, 2025 · 6 min read
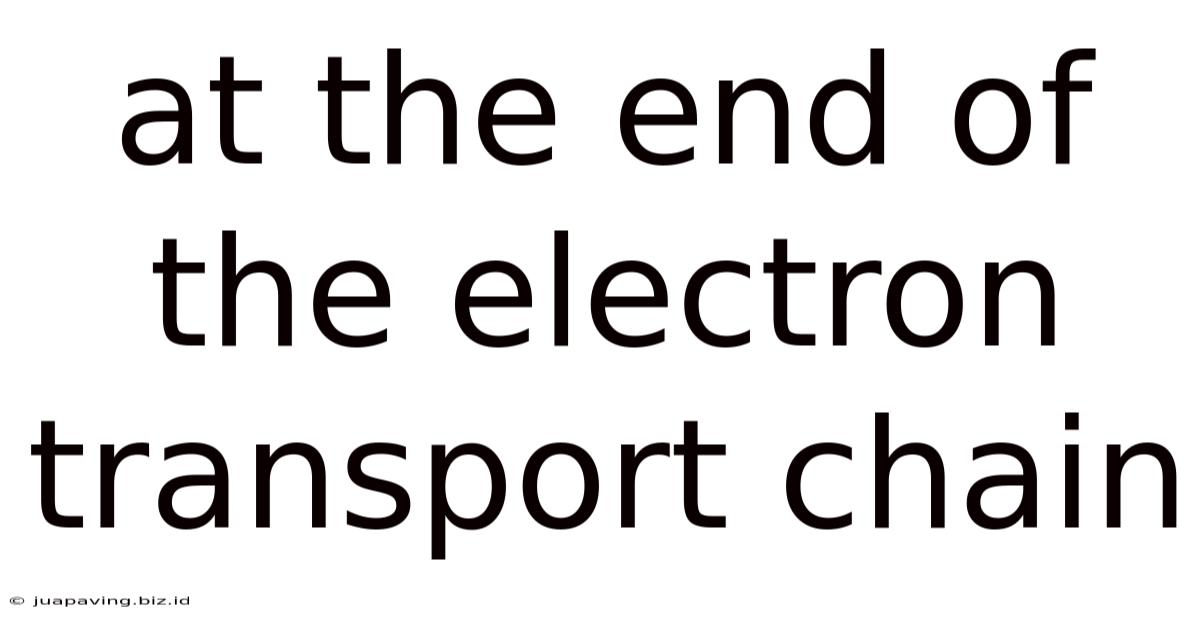
Table of Contents
At the End of the Electron Transport Chain: Water, Proton Gradient, and the Energetic Culmination of Cellular Respiration
The electron transport chain (ETC), a vital component of cellular respiration, is a marvel of biological engineering. This intricate series of protein complexes embedded within the inner mitochondrial membrane orchestrates the controlled release of energy from electrons, ultimately driving the synthesis of ATP, the cell's primary energy currency. But what happens at the very end of this remarkable chain? The answer is multifaceted, involving the formation of water, the establishment of a proton gradient, and the culmination of the energy-harvesting process. Understanding this final stage is key to comprehending the overall efficiency and importance of cellular respiration.
The Final Electron Acceptor: Oxygen's Crucial Role
The electron transport chain is essentially a carefully orchestrated electron "relay race." Electrons, initially energized from the breakdown of glucose in glycolysis and the citric acid cycle, are passed along a series of increasingly electronegative protein complexes (Complexes I-IV). This electron transfer releases energy, which is then harnessed to pump protons (H+) across the inner mitochondrial membrane, creating a proton gradient. However, this relay race needs a final destination – a molecule capable of accepting the electrons at the end of the chain. This crucial role is fulfilled by oxygen (O2).
Oxygen, owing to its high electronegativity, acts as the terminal electron acceptor. This means it has a very strong affinity for electrons and readily accepts them at the end of the ETC. Without oxygen, the electron transport chain would come to a standstill, severely impacting ATP production. This is precisely why oxygen is essential for aerobic respiration.
The Reaction at Complex IV: Forming Water
The final complex in the ETC, Complex IV (cytochrome c oxidase), catalyzes the reduction of oxygen to water. This is a crucial step, as it prevents the build-up of reactive oxygen species (ROS), which can damage cellular components. The reaction can be summarized as follows:
4e- + 4H+ + O2 → 2H2O
Four electrons (from cytochrome c), four protons (from the mitochondrial matrix), and one oxygen molecule combine to form two molecules of water. This reaction is highly exergonic, meaning it releases a significant amount of energy. However, this energy isn't directly used for ATP synthesis at this point. Instead, its primary role lies in driving the proton pumping activity of Complex IV.
The Proton Gradient: The Driving Force Behind ATP Synthesis
The energy released throughout the ETC is primarily used to pump protons from the mitochondrial matrix across the inner mitochondrial membrane into the intermembrane space. This creates a proton gradient, characterized by a higher concentration of protons in the intermembrane space compared to the matrix. This gradient is not just a concentration difference; it also represents an electrochemical gradient, as the positive charge of the protons contributes to a membrane potential.
This electrochemical proton gradient stores potential energy. It's this stored energy that drives the synthesis of ATP through chemiosmosis. Protons flow down their electrochemical gradient, back into the mitochondrial matrix, through a specialized enzyme called ATP synthase.
ATP Synthase: The Molecular Turbine
ATP synthase acts like a molecular turbine, utilizing the energy of the proton flow to rotate a central stalk. This rotation drives conformational changes within the enzyme, causing the synthesis of ATP from ADP and inorganic phosphate (Pi). This process is highly efficient, converting the potential energy of the proton gradient into the chemical energy stored in ATP.
The precise number of ATP molecules produced per electron pair passing through the ETC is still a subject of debate, with estimates ranging from 2.5 to 3 ATP molecules. Nevertheless, it's clear that the proton gradient, established at the end of the electron transport chain through oxygen reduction, is the crucial intermediate that links electron transport to ATP synthesis.
Beyond ATP Synthesis: Other Functions of the Proton Gradient
The proton gradient generated at the end of the electron transport chain doesn't exclusively fuel ATP synthesis. It plays a crucial role in several other cellular processes, including:
-
Transport of Metabolites: The gradient drives the active transport of various metabolites across the inner mitochondrial membrane, ensuring the efficient import of necessary substrates and export of waste products.
-
Regulation of Mitochondrial Function: The proton gradient contributes to the regulation of mitochondrial metabolism and the overall cellular energy balance. Changes in the gradient can act as signals to alter metabolic pathways.
-
Mitochondrial pH homeostasis: The pumping and subsequent flow of protons across the membrane influence the pH balance within the mitochondria and the surrounding cytoplasm, maintaining optimal conditions for cellular processes.
Reactive Oxygen Species (ROS) and Antioxidant Defenses
While oxygen is essential as the terminal electron acceptor, its reduction isn't always perfect. The ETC can sometimes leak electrons, leading to the formation of reactive oxygen species (ROS), such as superoxide radicals (O2•−), hydrogen peroxide (H2O2), and hydroxyl radicals (•OH). These ROS are highly reactive and can damage cellular components, including lipids, proteins, and DNA. Consequently, cells have evolved sophisticated antioxidant defense mechanisms to neutralize ROS and mitigate their harmful effects.
These defense mechanisms include enzymes like superoxide dismutase (SOD), which converts superoxide radicals to hydrogen peroxide, and catalase, which breaks down hydrogen peroxide into water and oxygen. Cells also utilize non-enzymatic antioxidants like glutathione and vitamin C to scavenge ROS.
The balance between ROS production and antioxidant defense is crucial for maintaining cellular health. Imbalance, with excessive ROS production, can lead to oxidative stress, which is implicated in various diseases, including cancer, aging, and neurodegenerative disorders.
The Efficiency of the Electron Transport Chain
The electron transport chain is remarkably efficient in harnessing the energy released from electron transfer. A significant portion of the energy from glucose oxidation is conserved in the form of ATP. While the precise efficiency varies depending on several factors, a significant amount of the energy initially available in glucose is captured during oxidative phosphorylation.
This remarkable efficiency underscores the elegance and precision of the biological machinery involved in cellular respiration. The carefully orchestrated electron transfer, proton pumping, and subsequent ATP synthesis represent a testament to the power of evolutionary adaptation.
Clinical Implications and Disease
Dysfunctions in the electron transport chain can have severe consequences, leading to a range of clinical conditions. Defects in the individual complexes or related proteins can result in mitochondrial diseases. These diseases are characterized by a wide spectrum of symptoms, depending on the specific defect and the affected tissues. Some examples include:
-
Leigh syndrome: A severe neurological disorder often caused by mutations in genes encoding ETC components.
-
Myoclonic epilepsy with ragged-red fibers (MERRF): Characterized by muscle weakness, epilepsy, and other neurological problems, often linked to mitochondrial DNA mutations affecting the ETC.
-
Mitochondrial myopathies: These affect muscles, causing weakness and fatigue.
The study of mitochondrial diseases continues to provide valuable insights into the intricate workings of the ETC and the importance of maintaining its proper function for cellular health.
Conclusion: A Symphony of Energy Conversion
The end of the electron transport chain isn't a simple endpoint; it's a culmination of a precisely orchestrated series of events. The reduction of oxygen to water, the establishment of a proton gradient, and the subsequent synthesis of ATP represent a breathtaking example of biological energy conversion. Understanding this final stage is vital not only for comprehending cellular respiration but also for appreciating the complexities of cellular life and the implications of disruptions to this crucial process. From the generation of water, the driving force of chemiosmosis, to the delicate balance between ROS production and antioxidant defense, the end of the electron transport chain stands as a testament to the sophisticated machinery of life itself.
Latest Posts
Latest Posts
-
How Tall Is 35 Inches In Feet
May 13, 2025
-
Snake Is Herbivores Carnivores Or Omnivores
May 13, 2025
-
What Is 35 Out Of 40
May 13, 2025
-
Low Level High Level Programming Languages
May 13, 2025
-
Solution Containing Maximum Amount Of Solute
May 13, 2025
Related Post
Thank you for visiting our website which covers about At The End Of The Electron Transport Chain . We hope the information provided has been useful to you. Feel free to contact us if you have any questions or need further assistance. See you next time and don't miss to bookmark.