As Temperatire Increases What Happens To The Condutor
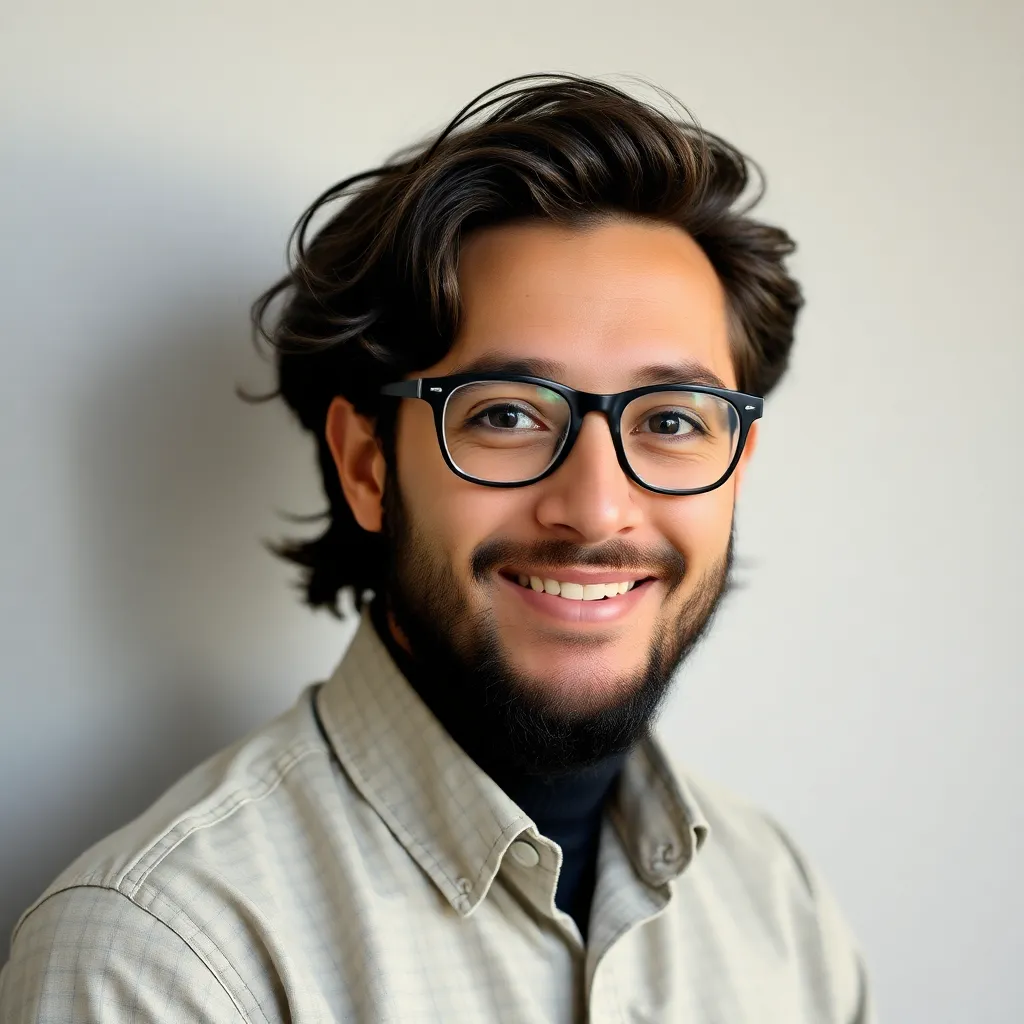
Juapaving
Apr 26, 2025 · 5 min read

Table of Contents
As Temperature Increases: What Happens to a Conductor?
The relationship between temperature and the conductivity of a material, particularly conductors, is a fundamental concept in physics and engineering. Understanding this relationship is crucial in numerous applications, from designing efficient power grids to developing advanced electronic devices. This article delves deep into the effects of increasing temperature on the conductivity of conductors, exploring the underlying mechanisms, the quantitative relationships, and the practical implications.
The Basics: Conductivity and Temperature
Conductivity, in the context of electrical engineering, refers to a material's ability to allow the flow of electric current. Conductors, by definition, exhibit high conductivity, meaning electrons can move relatively freely through their atomic structure. This freedom of electron movement is directly influenced by temperature.
The Atomic Dance: Electron Mobility and Thermal Energy
At absolute zero (-273.15°C or 0 Kelvin), the atoms in a conductor are essentially stationary. Electrons, while still present, are tightly bound to their respective atoms and have limited mobility. As temperature increases, the atoms gain kinetic energy and begin to vibrate more vigorously. This increased atomic vibration interferes with the free movement of electrons, leading to:
- Increased scattering: The electrons, while attempting to move through the conductor, collide more frequently with the vibrating atoms. These collisions impede their progress, thus reducing the overall flow of current.
- Reduced mean free path: The average distance an electron travels before colliding with an atom (the mean free path) decreases. Shorter mean free paths translate directly to lower conductivity.
- Higher resistivity: Resistivity is the inverse of conductivity. As the temperature rises and electron mobility decreases, the resistivity of the conductor increases.
The Mathematical Relationship: Linear and Non-Linear Behavior
The relationship between temperature and resistivity for many conductors can be approximated by a linear equation over a certain temperature range:
ρ(T) = ρ₀[1 + α(T - T₀)]
Where:
- ρ(T) is the resistivity at temperature T.
- ρ₀ is the resistivity at a reference temperature T₀ (often 20°C or 293.15 K).
- α is the temperature coefficient of resistivity, a material-specific constant that indicates how much the resistivity changes per degree Celsius.
This linear relationship holds reasonably well for many metals over a limited temperature range. However, it's crucial to remember that this is an approximation. At very high temperatures or low temperatures near absolute zero, the relationship becomes non-linear and more complex.
Deviation from Linearity: High and Low Temperature Regimes
At extremely high temperatures, the linear approximation breaks down due to several factors:
- Phase transitions: Some materials undergo phase transitions (e.g., melting) at high temperatures, drastically altering their atomic structure and, consequently, their conductivity.
- Lattice defects: High temperatures can lead to increased formation of lattice defects (imperfections in the crystal structure), further scattering electrons and reducing conductivity.
- Thermal ionization: At extremely high temperatures, electrons might gain enough energy to become thermally ionized, leaving atoms and further complicating the conductivity behaviour.
At very low temperatures (approaching absolute zero), quantum mechanical effects become dominant. Many conductors exhibit a phenomenon called superconductivity, where their resistivity drops to zero. This dramatic change in behavior is not described by the linear equation mentioned earlier.
Types of Conductors and Their Temperature Dependence
Different types of conductors exhibit varying degrees of sensitivity to temperature changes. Let's examine some common examples:
Metals: The Usual Suspects
Metals are the most common conductors. Their conductivity stems from the presence of free electrons in their outer shells. As temperature increases, the increased atomic vibrations lead to a linear increase in resistivity, as described earlier. However, the magnitude of this increase varies depending on the specific metal. Copper, for instance, has a relatively low temperature coefficient of resistivity compared to some other metals.
Semiconductors: A Different Story
Semiconductors are materials with intermediate conductivity between conductors and insulators. Their behavior is fundamentally different from metals regarding temperature dependence. In semiconductors, increasing the temperature increases the number of charge carriers (electrons and holes), leading to an increase in conductivity. This contrasts sharply with metals where increased temperature decreases conductivity. This temperature dependence is exploited in many semiconductor devices.
Superconductors: The Zero-Resistance Zone
Superconductors exhibit the remarkable property of having zero electrical resistance below a critical temperature (Tc). Below Tc, they can carry large currents without energy loss. This phenomenon is a purely quantum mechanical effect and is not explained by classical physics. The critical temperature varies significantly depending on the material. Some superconductors have extremely low critical temperatures, requiring cryogenic cooling, while others possess higher critical temperatures, making them potentially more practical for various applications.
Practical Implications: From Power Lines to Electronics
The temperature dependence of conductor resistivity has significant practical implications in various fields:
Power Transmission and Distribution:
The resistance of power lines increases with temperature, resulting in energy loss as heat. This loss is proportional to the square of the current and the resistance. Efficient power grid design accounts for this temperature dependence, considering factors like ambient temperature and current load.
Electronic Devices:
In electronic devices, the temperature dependence of resistance is crucial in designing and optimizing performance. For example, temperature sensors often utilize the temperature-sensitive resistance of certain materials to measure temperature accurately.
High-Temperature Applications:
In applications involving high temperatures, selecting appropriate conductors with low temperature coefficients of resistivity and high melting points is essential. Materials used in high-temperature furnaces or industrial heating elements need to withstand the thermal stress and maintain conductivity.
Conclusion: A Dynamic Relationship
The relationship between temperature and the conductivity of a conductor is a complex and multifaceted topic. While a linear approximation can be helpful in many situations, it's crucial to understand its limitations. Understanding the underlying physical mechanisms and considering the specific material properties is essential for designing and operating various electrical and electronic systems effectively. Whether it's managing energy losses in power grids or designing sensitive electronic components, accounting for temperature's impact on conductor resistivity is crucial for reliable and efficient performance. Further research continues to refine our understanding of this dynamic relationship and to explore new materials with tailored temperature-dependent conductivity for specific applications.
Latest Posts
Latest Posts
-
What Is Square Root Of 20
Apr 26, 2025
-
Is Every Rational Number Is An Integer
Apr 26, 2025
-
What Is The Difference Between An Atom And A Ion
Apr 26, 2025
-
Words That Begin With A W
Apr 26, 2025
-
What Are The Three Major Differences Between Dna And Rna
Apr 26, 2025
Related Post
Thank you for visiting our website which covers about As Temperatire Increases What Happens To The Condutor . We hope the information provided has been useful to you. Feel free to contact us if you have any questions or need further assistance. See you next time and don't miss to bookmark.