Alternative Forms Of A Gene Are Known As
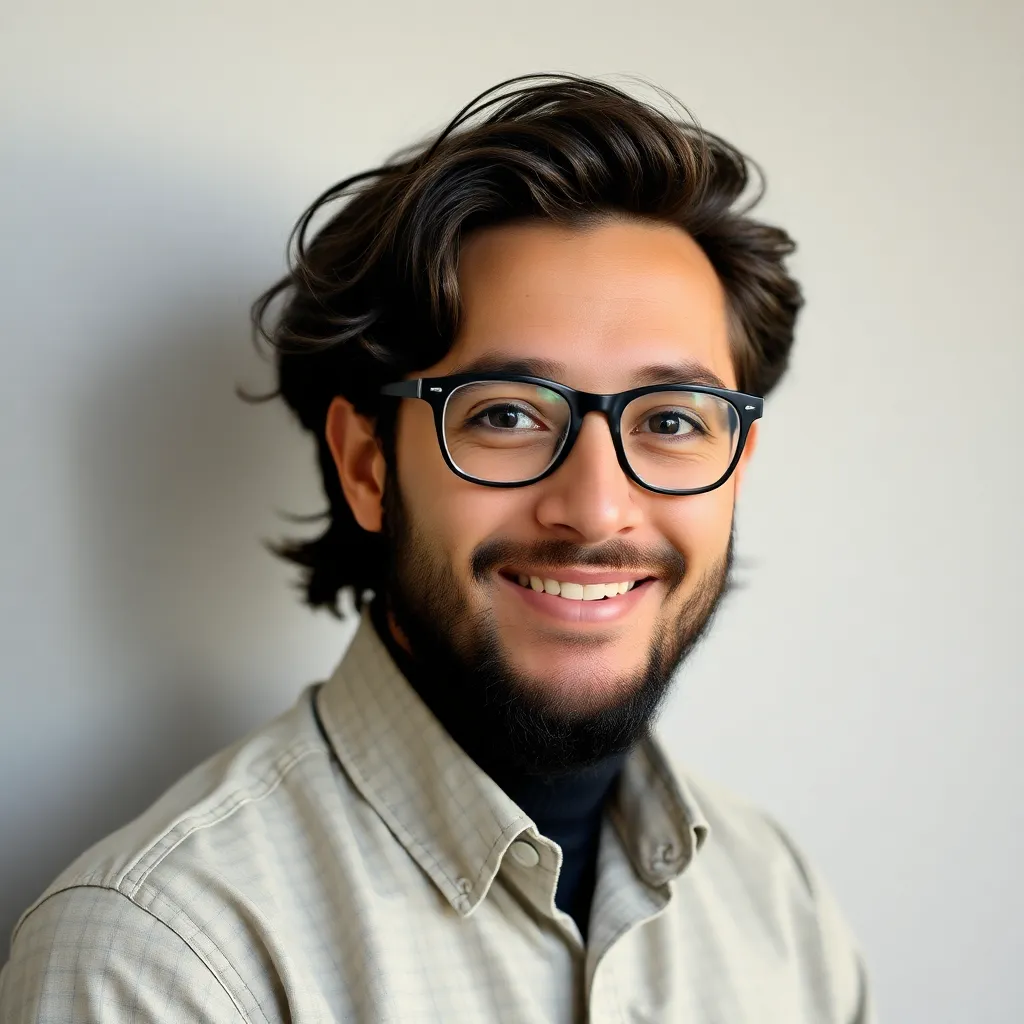
Juapaving
May 13, 2025 · 7 min read
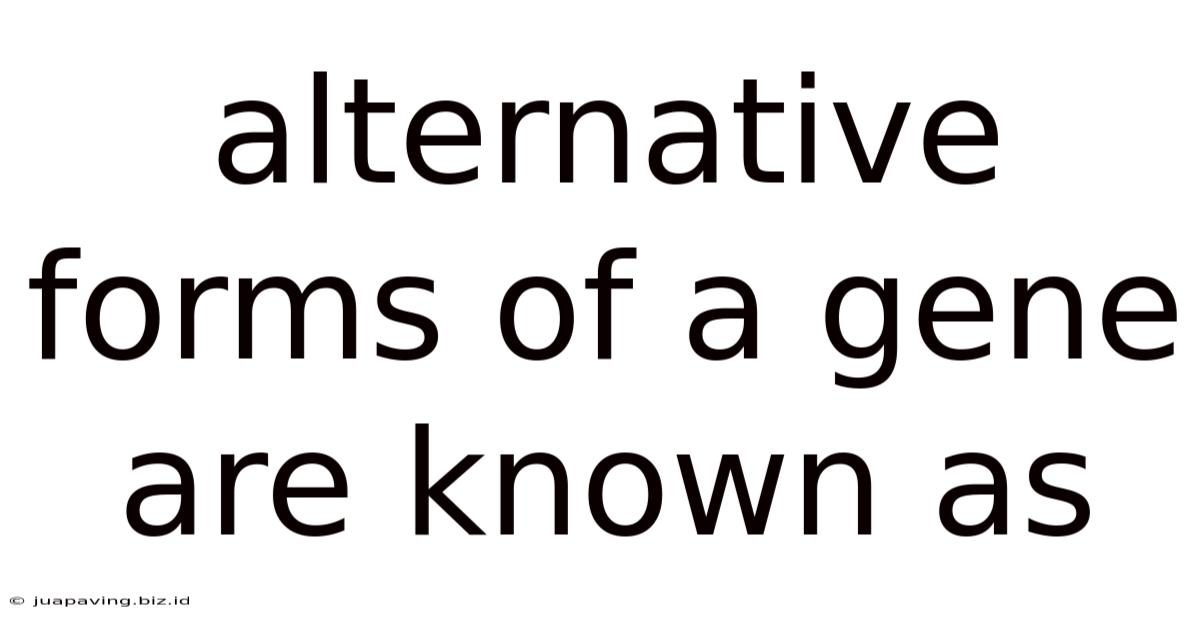
Table of Contents
Alternative Forms of a Gene are Known as Alleles: A Deep Dive into Genetics
Alternative forms of a gene are known as alleles. Understanding alleles is fundamental to grasping the complexities of heredity, genetic variation, and the mechanisms driving evolution. This comprehensive guide delves into the world of alleles, exploring their significance in various genetic contexts and their impact on observable traits.
What are Alleles?
At the most basic level, a gene is a specific sequence of DNA that provides the instructions for building a particular protein or performing a specific function within an organism. Alleles are different versions of the same gene. They occupy the same position, or locus, on homologous chromosomes. These homologous chromosomes are paired chromosomes, one inherited from each parent. Since each gene has a specific location on a chromosome, different alleles of a gene will be found at the same locus on each chromosome in a homologous pair.
For example, consider a gene responsible for eye color. This gene might have multiple alleles, some coding for brown eyes, others for blue eyes, green eyes, or even hazel eyes. Each individual inherits two alleles for each gene – one from their mother and one from their father. These two alleles, together, determine the individual's phenotype (observable characteristics) for that particular trait.
Types of Alleles:
Alleles can be classified into several categories based on their dominance relationships:
-
Dominant Alleles: A dominant allele exerts its effect even when paired with a different allele (a recessive allele). It masks the effect of the recessive allele. We often represent dominant alleles with a capital letter (e.g., 'B' for brown eyes).
-
Recessive Alleles: A recessive allele only manifests its effect when paired with another identical recessive allele. Its effect is masked by a dominant allele. We typically represent recessive alleles with a lowercase letter (e.g., 'b' for blue eyes).
-
Codominant Alleles: Codominant alleles both express themselves fully in the heterozygote (an individual with two different alleles). Neither allele masks the other. A classic example is the ABO blood group system, where alleles for A and B are both expressed in individuals with type AB blood.
-
Incomplete Dominance: In incomplete dominance, neither allele is completely dominant over the other. The heterozygote displays an intermediate phenotype, a blend of the phenotypes associated with each allele. For example, if a red flower (RR) is crossed with a white flower (WW), the resulting offspring (RW) might have pink flowers.
Genotype and Phenotype: The Expression of Alleles
The combination of alleles an individual possesses for a specific gene is called their genotype. The observable characteristics resulting from this genotype are known as the phenotype. The relationship between genotype and phenotype is not always straightforward.
For example:
-
Homozygous Dominant: An individual with two identical dominant alleles (e.g., BB for brown eyes) is homozygous dominant. Their phenotype will reflect the dominant trait.
-
Homozygous Recessive: An individual with two identical recessive alleles (e.g., bb for blue eyes) is homozygous recessive. Their phenotype will reflect the recessive trait.
-
Heterozygous: An individual with two different alleles (e.g., Bb for brown eyes, where B is dominant and b is recessive) is heterozygous. Their phenotype will usually reflect the dominant trait, but the recessive allele is still present in their genetic makeup.
The Significance of Alleles:
The existence of multiple alleles for a single gene is crucial for several reasons:
-
Genetic Diversity: Allelic variation is the foundation of genetic diversity within a population. This diversity is essential for adaptation to changing environments and for the long-term survival of a species. Without allelic variation, populations would be less resilient to environmental pressures, diseases, and other challenges.
-
Evolutionary Processes: Natural selection acts upon the phenotypic variation created by different alleles. Alleles that confer advantageous traits in a particular environment are more likely to be passed on to future generations, leading to evolutionary change. This is a key mechanism driving the evolution of species.
-
Understanding Genetic Diseases: Many genetic diseases are caused by the presence of specific alleles. Understanding the different alleles associated with these diseases is critical for diagnosis, genetic counseling, and the development of potential treatments. For instance, cystic fibrosis is caused by a recessive allele, meaning individuals need to inherit two copies of this allele to develop the disease.
-
Personalized Medicine: The increasing understanding of individual allelic variation is revolutionizing the field of medicine. Personalized medicine aims to tailor medical treatments to an individual's unique genetic makeup, improving the effectiveness and safety of therapies. Knowing an individual's alleles can help predict their response to certain drugs and guide treatment decisions.
-
Agriculture and Biotechnology: Allelic variation is also exploited in agriculture to improve crop yields, disease resistance, and nutritional content. Plant breeders utilize techniques such as selective breeding and genetic engineering to select and combine favorable alleles. This leads to the development of high-yielding and disease-resistant crops.
Beyond Simple Dominance and Recessive Relationships:
While the simple dominant-recessive model is helpful for understanding basic genetic principles, many genes exhibit more complex inheritance patterns. These complexities arise from the interactions between multiple genes, environmental influences, and epigenetic modifications.
Epigenetics and Gene Expression:
Epigenetics refers to heritable changes in gene expression that do not involve alterations to the underlying DNA sequence. Epigenetic modifications, such as DNA methylation and histone modification, can influence how readily genes are transcribed and translated into proteins. These modifications can affect the expression of alleles, leading to phenotypic variation even in individuals with the same genotype. This highlights the intricate interplay between genes and their environment in shaping observable traits.
Pleiotropy: One Gene, Multiple Effects:
Some genes can influence multiple seemingly unrelated traits, a phenomenon known as pleiotropy. A single allele can have cascading effects on various aspects of an organism's phenotype. Understanding pleiotropy is crucial for comprehending the complexity of gene function and its implications for health and disease. For example, a single gene might influence both eye color and the risk of certain types of cancer.
Polygenic Inheritance: Traits Influenced by Multiple Genes:
Many complex traits, such as height, skin color, and intelligence, are not determined by a single gene but rather by the combined effects of multiple genes. This is known as polygenic inheritance. Each gene involved may have multiple alleles, contributing to a wide range of phenotypic variation. The interplay of these multiple genes makes predicting the phenotype from the genotype challenging.
Analyzing Alleles: Techniques and Applications
Several techniques are used to study and analyze alleles:
-
Polymerase Chain Reaction (PCR): PCR allows for the amplification of specific DNA sequences, making it easier to study individual alleles.
-
DNA Sequencing: DNA sequencing determines the precise order of nucleotides in a DNA molecule, allowing for the identification of specific alleles. Next-generation sequencing (NGS) technologies have significantly advanced our ability to analyze a large number of alleles simultaneously.
-
Restriction Fragment Length Polymorphism (RFLP): RFLP is a technique that uses restriction enzymes to cut DNA at specific sites. Variations in DNA sequences between alleles can lead to different restriction fragment lengths, enabling the identification of specific alleles.
-
Microarray Analysis: Microarrays allow for the simultaneous analysis of thousands of genes and alleles, providing a comprehensive view of an organism's genetic makeup.
These techniques are used in a variety of applications, including:
-
Genetic testing: Identifying specific alleles associated with genetic diseases.
-
Forensic science: Using DNA fingerprinting to identify individuals.
-
Agriculture: Developing improved crop varieties.
-
Evolutionary biology: Studying the patterns of genetic variation in populations.
Conclusion: The Ubiquitous Role of Alleles
Alternative forms of a gene, known as alleles, are fundamental building blocks of genetics. Their existence underpins genetic variation, evolutionary processes, and the understanding of a myriad of biological phenomena. The study of alleles continues to evolve, driven by advancements in genomics and molecular biology. From understanding genetic diseases to developing personalized medicine and improving agricultural practices, the significance of alleles in various fields remains undeniable and continues to shape our understanding of life itself. Further research into allelic interactions and their regulatory mechanisms will undoubtedly unlock further insights into the complexities of life and provide valuable tools for addressing critical global challenges.
Latest Posts
Latest Posts
-
What Type Of Epithelium Lines The Kidney Tubules
May 13, 2025
-
Is Baking Soda Acid Base Or Neutral
May 13, 2025
-
Five Letter Words With E R
May 13, 2025
-
Major Rank In Indian Army Salary
May 13, 2025
-
Difference Between Combinational And Sequential Circuit
May 13, 2025
Related Post
Thank you for visiting our website which covers about Alternative Forms Of A Gene Are Known As . We hope the information provided has been useful to you. Feel free to contact us if you have any questions or need further assistance. See you next time and don't miss to bookmark.