A Sound Wave Is An Example Of A Longitudinal Wave.
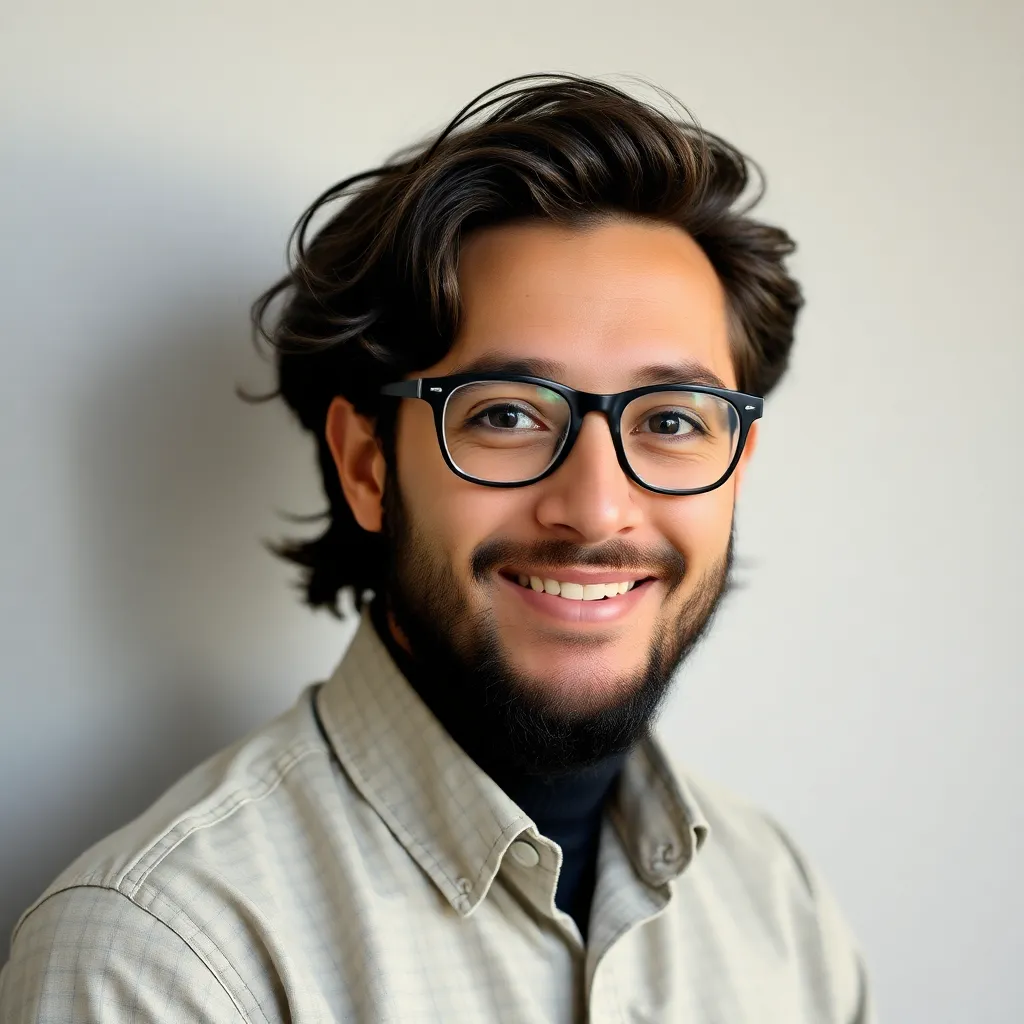
Juapaving
Apr 04, 2025 · 5 min read
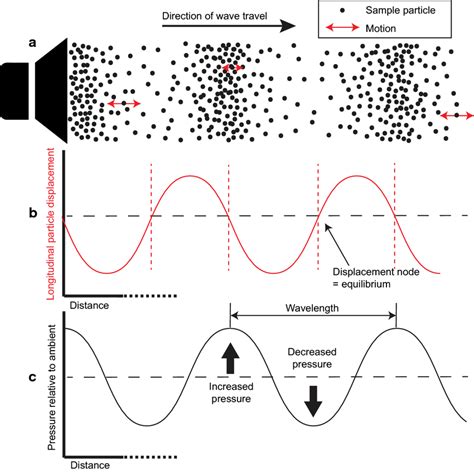
Table of Contents
A Sound Wave is an Example of a Longitudinal Wave: A Deep Dive
Sound, a ubiquitous phenomenon shaping our experience of the world, travels as a longitudinal wave. Understanding this fundamental characteristic unlocks a deeper appreciation of acoustics, music, and even medical imaging. This article delves into the physics of sound waves, explaining their longitudinal nature, contrasting them with transverse waves, exploring their properties, and touching upon their applications.
Understanding Waves: Transverse vs. Longitudinal
Before diving into the specifics of sound, let's establish a clear understanding of wave types. Waves are disturbances that transfer energy through a medium without transferring matter. There are two primary classifications:
Transverse Waves:
In transverse waves, the particles of the medium oscillate perpendicular (at right angles) to the direction of wave propagation. Think of a wave rippling across the surface of a pond. The water molecules move up and down, while the wave itself travels horizontally. Examples include:
- Light waves: Electromagnetic waves that don't require a medium to travel.
- Waves on a stringed instrument: The string vibrates perpendicular to the direction the wave travels along the string.
- Seismic S-waves: Secondary waves generated during earthquakes.
Longitudinal Waves:
In longitudinal waves, the particles of the medium oscillate parallel to the direction of wave propagation. Imagine pushing and pulling a spring: the coils move back and forth along the spring's length, and the compression and rarefaction travel along the spring. This is analogous to how sound travels.
Sound Waves: A Longitudinal Phenomenon
Sound waves are a classic example of longitudinal waves. They propagate through a medium (such as air, water, or solids) by compressing and rarefying the particles of that medium.
Compression and Rarefaction: The Mechanics of Sound
When a sound source, like a vibrating speaker cone, moves outward, it compresses the air molecules in front of it, creating a region of high pressure called a compression. As the source moves back inward, it leaves a region of low pressure called a rarefaction. This cycle of compression and rarefaction creates a traveling wave that propagates outward from the source.
The Role of the Medium:
The speed of sound varies depending on the medium it travels through. Sound travels faster in denser mediums because the particles are closer together, allowing for quicker transmission of the compression and rarefaction pulses. For example, sound travels faster in water than in air, and even faster in solids. A vacuum, lacking a medium, cannot transmit sound.
Key Properties of Sound Waves
Several key properties define and characterize sound waves:
1. Wavelength (λ):
The wavelength is the distance between two consecutive compressions (or rarefactions). It's inversely proportional to frequency. A longer wavelength corresponds to a lower frequency, and vice versa.
2. Frequency (f):
The frequency represents the number of compressions (or rarefactions) that pass a given point per second. It's measured in Hertz (Hz), where 1 Hz equals one cycle per second. Frequency determines the pitch of a sound; higher frequencies correspond to higher pitches.
3. Amplitude:
The amplitude of a sound wave represents the maximum displacement of particles from their equilibrium position. Amplitude is directly related to the intensity or loudness of the sound. A larger amplitude corresponds to a louder sound.
4. Speed (v):
The speed of a sound wave is determined by the properties of the medium it travels through. As mentioned earlier, denser mediums generally allow for faster sound propagation. The relationship between speed, frequency, and wavelength is given by the equation: v = fλ
.
Visualizing Longitudinal Waves:
While it can be challenging to visualize longitudinal waves compared to the more easily depicted transverse waves, several methods help illustrate the concept:
- Spring Model: Using a spring to demonstrate compression and rarefaction provides a clear analogy for how sound waves propagate.
- Graphical Representation: A graph plotting pressure (or particle displacement) against distance can represent the compressions and rarefactions of a longitudinal wave. The peaks represent compressions, and the troughs represent rarefactions.
- Software Simulations: Numerous physics simulation software packages allow for dynamic visualizations of longitudinal waves, offering a more interactive understanding.
Applications of Understanding Longitudinal Waves
The understanding of longitudinal waves, specifically sound waves, has far-reaching applications across various fields:
1. Acoustics:
Acoustics, the science of sound, relies heavily on understanding the properties of longitudinal waves. Design of concert halls, recording studios, and noise-cancellation technologies all leverage this knowledge.
2. Medical Imaging:
Ultrasound imaging utilizes high-frequency sound waves to create images of internal organs. The reflection of sound waves from different tissues allows for visualization without the use of ionizing radiation.
3. Sonar and Radar:
Sonar (sound navigation and ranging) uses sound waves to detect objects underwater, while radar (radio detection and ranging) uses electromagnetic waves (transverse) but operates on similar principles. Both rely on the emission of waves and the analysis of their reflections.
4. Music:
Musical instruments produce sound through the vibration of strings, air columns, or membranes. Understanding the properties of sound waves helps in designing instruments, tuning them, and analyzing the sounds they produce.
Distinguishing Longitudinal from Transverse Waves: A Summary
The key difference lies in the direction of particle oscillation relative to the wave's propagation direction. In transverse waves, particles move perpendicularly, while in longitudinal waves, they move parallel. This fundamental difference leads to variations in wave behavior and applications.
Conclusion:
Sound, a cornerstone of our sensory experience, is a testament to the power of longitudinal waves. By grasping the mechanics of compression and rarefaction, and the properties that characterize these waves, we unlock a deeper understanding of this fundamental phenomenon. From the intricacies of musical instruments to the sophisticated applications in medical imaging and sonar, the study of longitudinal sound waves reveals its profound impact on our world. Further exploration into the intricacies of wave interference, superposition, and Doppler effect will lead to even richer appreciation of this remarkable wave phenomenon. The study of sound waves is a continuously evolving field, with ongoing research pushing the boundaries of our understanding and leading to innovative applications.
Latest Posts
Latest Posts
-
32 Degrees Is What In Celsius
Apr 10, 2025
-
3 Inches Is How Many Centimeters
Apr 10, 2025
-
How Many Diagonals Does A Rectangle Have
Apr 10, 2025
-
What Are The Most Reactive Nonmetals On The Periodic Table
Apr 10, 2025
-
Find The Value Of Each Trigonometric Ratio
Apr 10, 2025
Related Post
Thank you for visiting our website which covers about A Sound Wave Is An Example Of A Longitudinal Wave. . We hope the information provided has been useful to you. Feel free to contact us if you have any questions or need further assistance. See you next time and don't miss to bookmark.