A Single Carbon Atom Can Form A Maximum Of
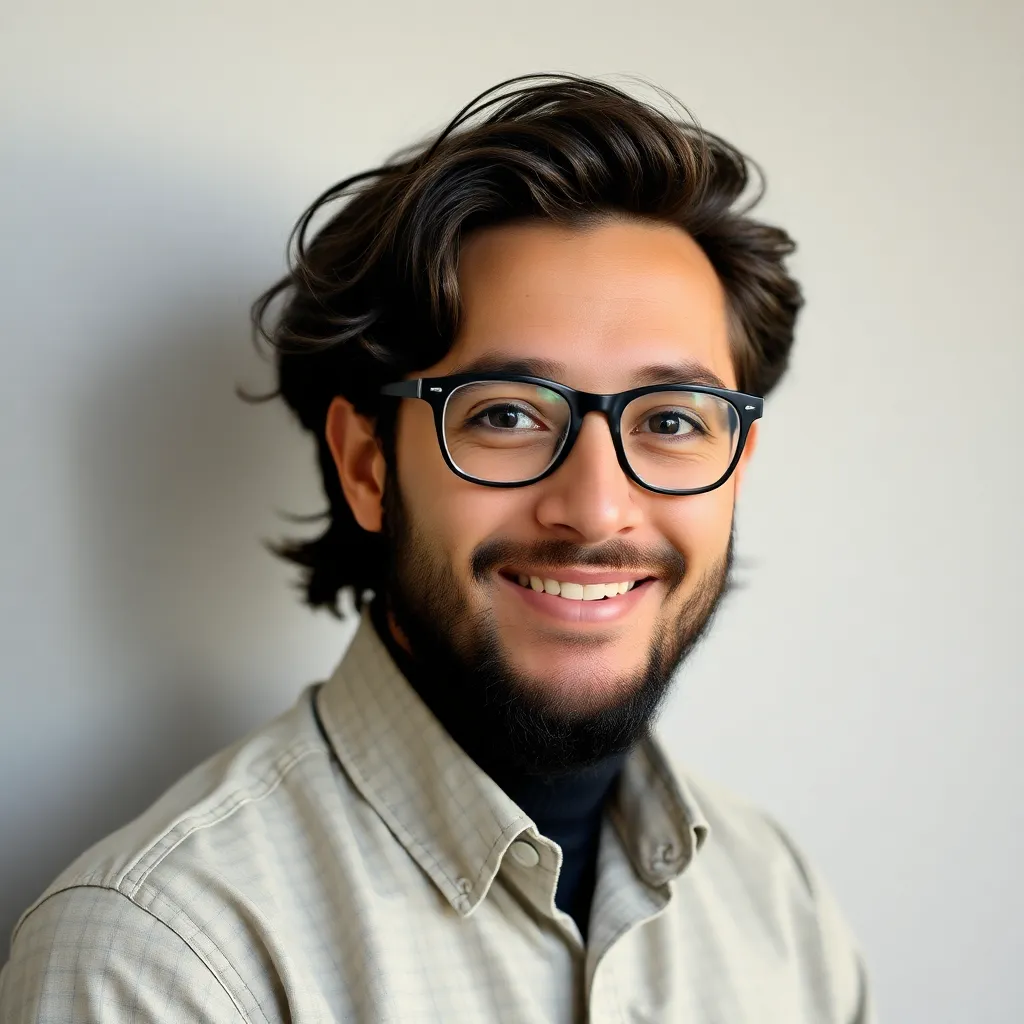
Juapaving
Apr 07, 2025 · 6 min read
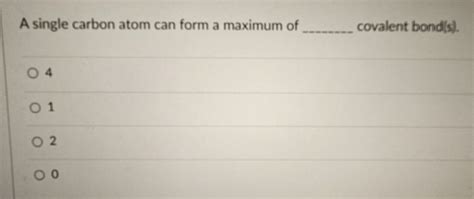
Table of Contents
A Single Carbon Atom Can Form a Maximum of Four Bonds: Exploring the Wonders of Carbon Chemistry
Carbon, the backbone of life and the foundation of countless materials, possesses a unique property that sets it apart from other elements: its unparalleled ability to form a vast array of molecules. This remarkable characteristic stems from its electron configuration and its capacity to form a maximum of four covalent bonds. Understanding this fundamental principle unlocks the door to comprehending the immense diversity and complexity of organic chemistry and materials science.
The Electron Configuration: The Key to Carbon's Bonding Prowess
At the heart of carbon's bonding capacity lies its electronic structure. A neutral carbon atom has six electrons, arranged in two shells: two electrons in the first shell (1s²) and four electrons in the second shell (2s²2p²). The first shell is completely filled, while the second shell holds only four electrons, leaving it incomplete. To achieve a stable electron configuration, similar to the inert gases (like neon and argon), carbon atoms strive to acquire a full octet—eight electrons in their outermost shell.
This pursuit of stability drives carbon atoms to form covalent bonds, sharing electrons with other atoms to complete their octet. Since carbon has four electrons in its outermost shell and needs four more to reach eight, it can form a maximum of four covalent bonds. This seemingly simple fact is the cornerstone of the extraordinary diversity of carbon-based molecules.
The Variety of Carbon Bonds: Single, Double, and Triple
The four bonds a carbon atom can form aren't always identical. Carbon exhibits versatility in its bonding, forming single, double, and triple bonds:
Single Bonds: The Foundation of Carbon Chains
A single bond involves the sharing of one pair of electrons between two atoms. In carbon, this forms a strong, relatively stable bond, allowing for the formation of long chains and branched structures. The simplest example is methane (CH₄), where a central carbon atom is bonded to four hydrogen atoms via four single bonds. These long chains and branched structures are fundamental to the structure of polymers, proteins, and many other organic molecules. The strength and stability of single bonds make them essential for building the backbone of complex molecules.
Double Bonds: Introducing Rigidity and Reactivity
A double bond consists of two pairs of shared electrons between two atoms. This creates a stronger bond than a single bond, resulting in shorter bond lengths and higher bond energies. Double bonds often introduce rigidity into a molecule, affecting its three-dimensional shape and its reactivity. Ethylene (C₂H₄), the simplest alkene, exemplifies a molecule containing a carbon-carbon double bond. The presence of a double bond significantly influences the molecule's chemical properties and reactivity, making it more susceptible to certain types of reactions.
Triple Bonds: Maximum Bond Strength and Linearity
A triple bond involves the sharing of three pairs of electrons between two atoms, creating the strongest and shortest bond between carbon atoms. This type of bond is highly reactive and usually restricts the molecule to a linear geometry. Acetylene (C₂H₂), the simplest alkyne, is a classic example. The triple bond's high energy makes acetylene a valuable fuel and a versatile building block in organic synthesis. The linear nature of triple bonds influences the overall shape and reactivity of molecules containing them.
Beyond the Basics: Hybrid Orbitals and Molecular Geometry
The simplistic picture of four separate bonds radiating from a carbon atom is an oversimplification. To fully understand the bonding in carbon, we must delve into the concept of hybrid orbitals. Carbon's four valence electrons occupy one s orbital and three p orbitals. However, in many carbon compounds, these orbitals hybridize to form new orbitals with different shapes and energies.
sp³ Hybridization: Tetrahedral Geometry
In sp³ hybridization, one s orbital and three p orbitals combine to form four equivalent sp³ hybrid orbitals, arranged in a tetrahedral geometry with bond angles of approximately 109.5°. This hybridization is common in alkanes like methane and ethane, where the carbon atoms are bonded to four other atoms with single bonds. The tetrahedral geometry is crucial for the three-dimensional structure of many organic molecules, influencing their properties and reactivity.
sp² Hybridization: Trigonal Planar Geometry
sp² hybridization involves the combination of one s orbital and two p orbitals, forming three equivalent sp² hybrid orbitals arranged in a trigonal planar geometry with bond angles of approximately 120°. The remaining p orbital is unhybridized and participates in the formation of a π (pi) bond, as seen in alkenes and carbonyl compounds. The planar geometry is essential for the understanding of reactivity in unsaturated organic molecules.
sp Hybridization: Linear Geometry
In sp hybridization, one s orbital and one p orbital combine to form two equivalent sp hybrid orbitals arranged linearly with a bond angle of 180°. The remaining two p orbitals form two π (pi) bonds, as observed in alkynes. The linear geometry impacts the reactivity and physical properties of alkynes.
The Implications of Carbon's Bonding: A World of Possibilities
The ability of a single carbon atom to form a maximum of four bonds, combined with its capacity for various bond types and hybrid orbital formations, has profound implications across diverse scientific fields:
Organic Chemistry: The Foundation of Life
The unparalleled versatility of carbon bonding is the foundation of organic chemistry, the study of carbon-containing compounds. From simple hydrocarbons to complex biomolecules like proteins, DNA, and carbohydrates, the vast array of carbon-based molecules underpins the structure and function of all living organisms. The ability of carbon to form long chains, branched structures, and rings leads to the immense diversity of organic molecules.
Materials Science: Creating Novel Materials
The unique properties of carbon are exploited in the development of numerous materials. Diamond, with its strong sp³ hybridized carbon network, is renowned for its hardness. Graphite, with its layered sp² hybridized structure, exhibits excellent conductivity. Fullerenes (like buckminsterfullerene, C₆₀) and carbon nanotubes, with their unique structures, offer exciting possibilities for future materials with exceptional electronic, mechanical, and optical properties. These materials exemplify the vast potential arising from the versatility of carbon bonding.
Nanotechnology: Building at the Atomic Level
Carbon's ability to form diverse structures is central to nanotechnology. Carbon nanotubes, graphene (a single layer of graphite), and fullerenes are investigated for their potential in electronics, medicine, and energy applications. Their unique electrical, mechanical, and chemical properties make them ideal building blocks for nanoscale devices and materials. Nanotechnology leverages carbon's unique bonding capabilities to create materials with unprecedented functionalities.
Pharmaceuticals: Designing Life-Saving Drugs
Carbon's versatility plays a vital role in the design and development of pharmaceuticals. Many drugs are based on carbon skeletons, with functional groups attached to modify their properties and biological activity. The specific arrangements of carbon atoms and functional groups determine a drug's effectiveness and its interaction with biological targets. Understanding carbon bonding is fundamental for the rational design of new drugs.
Conclusion: The Enduring Significance of Carbon's Bonding Capacity
The ability of a single carbon atom to form a maximum of four bonds is a cornerstone of chemistry and materials science. This seemingly simple fact underpins the astonishing diversity of organic molecules, the creation of novel materials, the advancements in nanotechnology, and the development of life-saving drugs. The study of carbon bonding continues to be a fertile ground for scientific discovery, promising a plethora of exciting advancements in the years to come. Understanding this fundamental concept is crucial for anyone seeking to explore the wonders of chemistry and its impact on our world.
Latest Posts
Latest Posts
-
Least Common Multiple Of 10 And 11
Apr 07, 2025
-
16 3x 5 10 4x 8 40
Apr 07, 2025
-
Is Force Increases On An Inclined Plane
Apr 07, 2025
-
An Area Of 640 Acres Is Equal To
Apr 07, 2025
-
What Is 59 In Roman Numerals
Apr 07, 2025
Related Post
Thank you for visiting our website which covers about A Single Carbon Atom Can Form A Maximum Of . We hope the information provided has been useful to you. Feel free to contact us if you have any questions or need further assistance. See you next time and don't miss to bookmark.