A Metal Crystallizes In A Face-centered Cubic Lattice
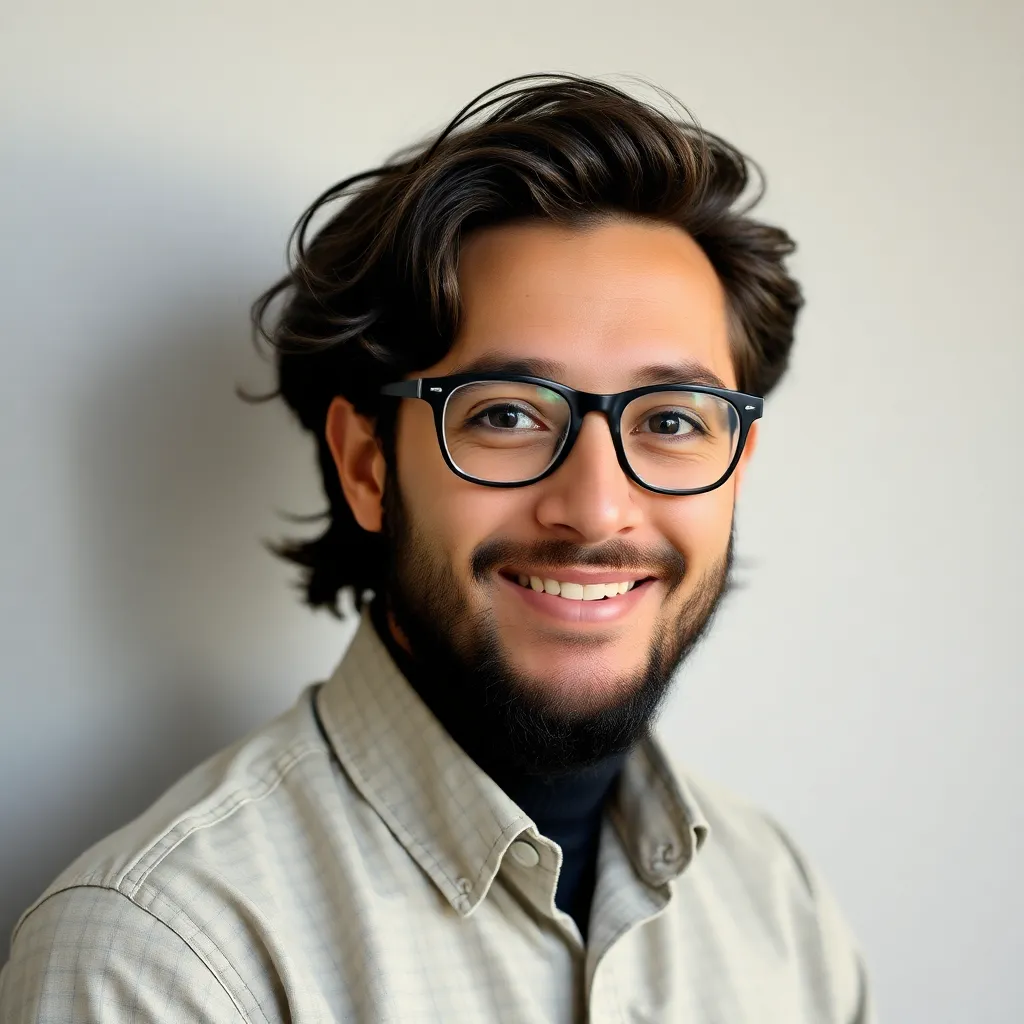
Juapaving
May 12, 2025 · 6 min read
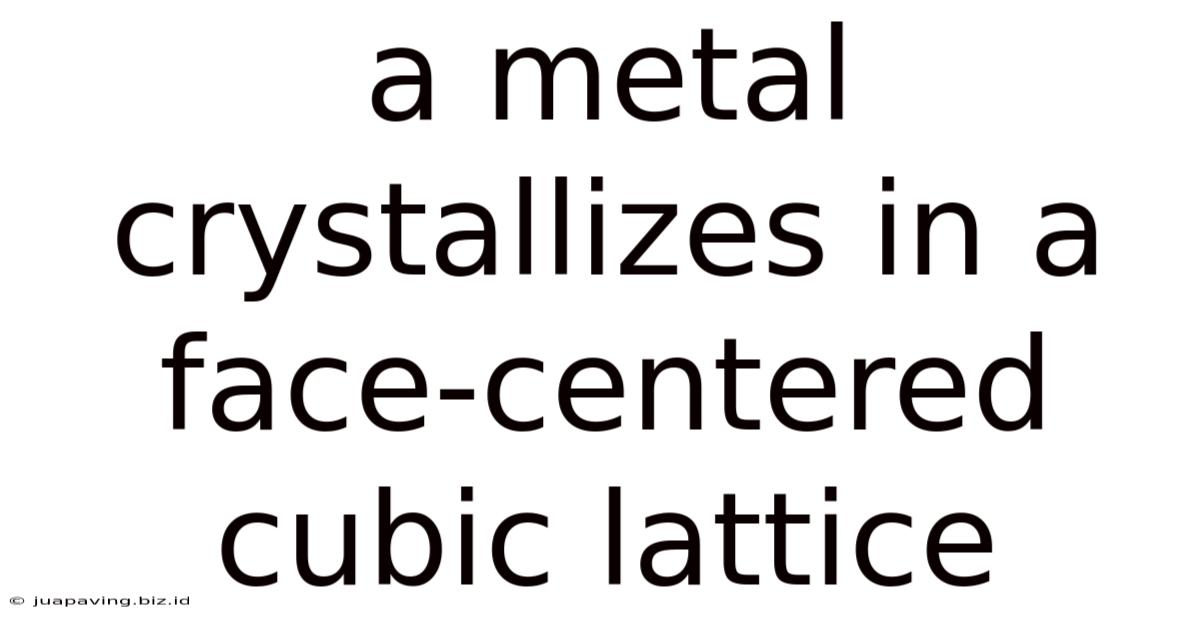
Table of Contents
A Metal Crystallizes in a Face-Centered Cubic Lattice: Unpacking the Structure and Properties
Metals, the foundational materials of countless technologies, often exhibit a fascinating internal structure at the atomic level. One common arrangement is the face-centered cubic (FCC) lattice, a highly ordered packing arrangement that significantly influences a metal's physical and chemical properties. This article delves into the intricacies of the FCC lattice, exploring its structure, characteristics, and implications for the behavior of metals.
Understanding the Face-Centered Cubic Lattice
The FCC lattice is a type of cubic crystal system where atoms are positioned at each of the eight corners of a cube and at the center of each of the six faces. This arrangement leads to a highly efficient packing density, maximizing the number of atoms within a given volume. Let's break down the key features:
Atomic Positions and Coordination Number
- Corner Atoms: Eight atoms are located at the corners of the cube, each contributing 1/8 of its volume to the unit cell.
- Face-Centered Atoms: Six atoms reside at the center of each face, each contributing 1/2 of its volume to the unit cell.
This arrangement results in a total of 4 atoms per unit cell (8 corner atoms x 1/8 + 6 face-centered atoms x 1/2 = 4 atoms).
The coordination number, representing the number of nearest neighbors surrounding a given atom, is 12 in an FCC lattice. Each atom is in contact with 12 other atoms, resulting in a tightly packed structure. This high coordination number contributes to the metal's strength and ductility.
Packing Efficiency and Atomic Radius
The FCC lattice boasts an exceptionally high packing efficiency, a measure of the volume occupied by atoms within the unit cell. In an FCC structure, the packing efficiency is approximately 74%, significantly higher than simple cubic (52%) and body-centered cubic (68%) structures. This high packing efficiency leads to denser metals with higher density compared to other crystal structures.
The relationship between the atomic radius (r) and the lattice parameter (a), the length of the cube's edge, in an FCC lattice is defined by:
a = 2√2r
This equation highlights the direct correlation between atomic size and the overall dimensions of the unit cell.
Slip Systems and Plastic Deformation
The FCC structure's characteristic close-packed planes and directions play a critical role in determining its mechanical properties, particularly its ability to undergo plastic deformation. Slip systems, which are combinations of specific crystallographic planes and directions along which dislocation movement occurs, are abundant in FCC metals. These numerous slip systems contribute to the high ductility and malleability often associated with FCC metals. The ease of dislocation movement along these planes allows for significant plastic deformation before fracture, a key characteristic that makes FCC metals suitable for applications requiring formability.
Metals Exhibiting FCC Structure
Many common and industrially significant metals crystallize in the FCC lattice. These include:
- Aluminum (Al): Widely used in aerospace, automotive, and packaging industries due to its lightweight nature, corrosion resistance, and good formability.
- Copper (Cu): An excellent electrical and thermal conductor, used extensively in electrical wiring, plumbing, and alloys.
- Nickel (Ni): A strong, corrosion-resistant metal used in various alloys, including stainless steel and superalloys.
- Gold (Au): Valued for its inertness, malleability, and luster, extensively used in jewelry and electronics.
- Silver (Ag): Another excellent electrical conductor, also used in jewelry, photography, and electronics.
- Platinum (Pt): A precious metal with high corrosion resistance, used in catalytic converters and jewelry.
- Lead (Pb): Although toxic, lead's malleability and resistance to corrosion have historically led to its use in various applications, though these are diminishing due to environmental concerns.
- γ-Iron (Austenite): A high-temperature allotrope of iron, crucial to the properties of stainless steel.
These examples underscore the prevalence of the FCC structure among metals with diverse applications.
Implications of FCC Structure on Material Properties
The FCC lattice structure profoundly impacts a metal's various properties.
Mechanical Properties
- Ductility and Malleability: The numerous slip systems in FCC metals contribute significantly to their high ductility and malleability, enabling them to undergo substantial plastic deformation before fracture. This is a key advantage in forming and shaping these metals into various components.
- Strength: While generally less strong than BCC metals at room temperature, FCC metals can exhibit high strength at elevated temperatures due to their ability to work-harden.
- Hardness: The close-packed nature of the FCC structure generally leads to moderate hardness. However, this can be significantly increased through various strengthening mechanisms like alloying and cold working.
- Toughness: The combination of ductility and strength contributes to a reasonable level of toughness in FCC metals, representing their ability to absorb energy before fracture.
Physical Properties
- Density: The high packing efficiency of the FCC structure results in high density compared to other crystal structures.
- Thermal Conductivity: FCC metals generally exhibit high thermal conductivity due to the efficient electron transport facilitated by the close-packed structure and delocalized electrons.
- Electrical Conductivity: Similar to thermal conductivity, the delocalized electrons in FCC metals contribute to high electrical conductivity, making them suitable for electrical applications.
- Melting Point: The strength of metallic bonds influences the melting point. While there is no direct relationship between the FCC structure and melting point, the nature of bonding in FCC metals often results in relatively high melting points.
Chemical Properties
The chemical properties of FCC metals are largely determined by their electronic configuration and the nature of their atomic bonding. However, the FCC structure itself doesn't directly influence chemical reactivity in a significant way. Corrosion resistance, for example, is largely determined by factors like the metal's position in the electrochemical series and the formation of protective oxide layers.
Alloying and its Effects on FCC Metals
Alloying, the process of adding other elements to a base metal, significantly alters the properties of FCC metals. Alloying can:
- Increase Strength: Alloying elements can impede dislocation motion, thereby enhancing the metal's strength.
- Improve Corrosion Resistance: Adding elements that form protective oxide layers enhances corrosion resistance.
- Modify Electrical Conductivity: Alloying can either increase or decrease electrical conductivity depending on the added element.
- Alter Ductility: Alloying can either improve or reduce ductility, depending on the specific alloying element and its concentration.
The careful selection of alloying elements allows for tailoring the properties of FCC metals to meet the specific requirements of different applications.
Advanced Techniques for Studying FCC Structures
Various advanced techniques are employed to study the structure and properties of FCC metals at the atomic level. These include:
- X-ray Diffraction (XRD): This technique utilizes the diffraction of X-rays by the crystal lattice to determine the lattice parameters and identify the crystal structure.
- Transmission Electron Microscopy (TEM): TEM provides high-resolution images of the crystal structure, revealing details about defects and dislocations.
- Scanning Electron Microscopy (SEM): SEM offers high-magnification images of the metal's surface, revealing details about its microstructure.
- Atom Probe Tomography (APT): APT is a powerful technique that can determine the three-dimensional atomic-scale composition of a material, providing crucial insights into alloying effects.
These techniques provide valuable information about the structure, defects, and composition of FCC metals, contributing to a deeper understanding of their properties.
Conclusion
The face-centered cubic lattice represents a significant organizational structure in the world of metals, influencing a wide range of properties relevant to engineering and material science. Its high packing efficiency, numerous slip systems, and suitability for alloying make FCC metals indispensable across diverse applications. Understanding the intricate relationship between structure, properties, and processing is crucial for developing advanced materials with tailored characteristics for future technologies. Further research and development focused on exploring the nuances of FCC metals will continue to unlock their potential and pave the way for innovations in various fields.
Latest Posts
Latest Posts
-
Which Lists The Correct Order Of Evolutionary History
May 12, 2025
-
What Is Inertia Measured In Units
May 12, 2025
-
Which Expression Is Represented By The Model
May 12, 2025
-
Blood Plasma Without Clotting Factors Is Called
May 12, 2025
-
Which Of The Following Is An Operating System
May 12, 2025
Related Post
Thank you for visiting our website which covers about A Metal Crystallizes In A Face-centered Cubic Lattice . We hope the information provided has been useful to you. Feel free to contact us if you have any questions or need further assistance. See you next time and don't miss to bookmark.